Adipocyte-released adipomes in Chagas cardiomyopathy: Impact on cardiac metabolic and immune regulation
- PMID: 38660407
- PMCID: PMC11039351
- DOI: 10.1016/j.isci.2024.109672
Adipocyte-released adipomes in Chagas cardiomyopathy: Impact on cardiac metabolic and immune regulation
Abstract
Chronic Trypanosoma cruzi infection leads to Chagas cardiomyopathy (CCM), with varying manifestations such as inflammatory hypertrophic cardiomyopathy, arrhythmias, and dilated cardiomyopathy. The factors responsible for the increasing risk of progression to CCM are not fully understood. Previous studies link adipocyte loss to CCM progression, but the mechanism triggering CCM pathogenesis remains unexplored. Our study uncovers that T. cruzi infection triggers adipocyte apoptosis, leading to the release of extracellular vesicles named "adipomes". We developed an innovative method to isolate intact adipomes from infected mice's adipose tissue and plasma, showing they carry unique lipid cargoes. Large and Small adipomes, particularly plasma-derived infection-associated L-adipomes (P-ILA), regulate immunometabolic signaling and induce cardiomyopathy. P-ILA treatment induces hypertrophic cardiomyopathy in wild-type mice and worsens cardiomyopathy severity in post-acute-infected mice by regulating adipogenic/lipogenic and mitochondrial functions. These findings highlight adipomes' pivotal role in promoting inflammation and impairing myocardial function during cardiac remodeling in CD.
Keywords: Biology experimental methods; Cell biology; Disease; Microbiology parasite.
© 2024 The Author(s).
Conflict of interest statement
The authors declare no competing interests.
Figures
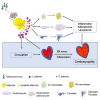
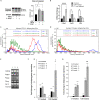
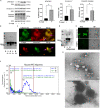
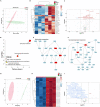
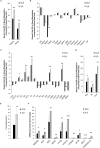
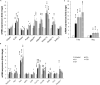
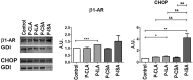
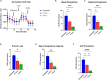
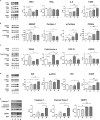
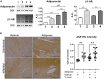
Similar articles
-
Diet Alters Serum Metabolomic Profiling in the Mouse Model of Chronic Chagas Cardiomyopathy.Dis Markers. 2019 Dec 20;2019:4956016. doi: 10.1155/2019/4956016. eCollection 2019. Dis Markers. 2019. PMID: 31949545 Free PMC article.
-
Inhibition of SREBP Improves Cardiac Lipidopathy, Improves Endoplasmic Reticulum Stress, and Modulates Chronic Chagas Cardiomyopathy.J Am Heart Assoc. 2020 Feb 4;9(3):e014255. doi: 10.1161/JAHA.119.014255. Epub 2020 Jan 24. J Am Heart Assoc. 2020. PMID: 31973605 Free PMC article.
-
PARP1-cGAS-NF-κB pathway of proinflammatory macrophage activation by extracellular vesicles released during Trypanosoma cruzi infection and Chagas disease.PLoS Pathog. 2020 Apr 21;16(4):e1008474. doi: 10.1371/journal.ppat.1008474. eCollection 2020 Apr. PLoS Pathog. 2020. PMID: 32315358 Free PMC article.
-
Extracellular Vesicles: Potential Role in Remote Signaling and Inflammation in Trypanosoma cruzi-Triggered Disease.Front Cell Dev Biol. 2021 Dec 20;9:798054. doi: 10.3389/fcell.2021.798054. eCollection 2021. Front Cell Dev Biol. 2021. PMID: 34988085 Free PMC article. Review.
-
The Oxidative Stress and Chronic Inflammatory Process in Chagas Disease: Role of Exosomes and Contributing Genetic Factors.Oxid Med Cell Longev. 2021 Dec 23;2021:4993452. doi: 10.1155/2021/4993452. eCollection 2021. Oxid Med Cell Longev. 2021. PMID: 34976301 Free PMC article. Review.
References
-
- Chadalawada S., Sillau S., Archuleta S., Mundo W., Bandali M., Parra-Henao G., Rodriguez-Morales A.J., Villamil-Gomez W.E., Suárez J.A., Shapiro L., et al. Risk of Chronic Cardiomyopathy Among Patients With the Acute Phase or Indeterminate Form of Chagas Disease: A Systematic Review and Meta-analysis. JAMA Netw. Open. 2020;3 doi: 10.1001/jamanetworkopen.2020.15072. - DOI - PMC - PubMed
-
- Nunes M.C.P., Beaton A., Acquatella H., Bern C., Bolger A.F., Echeverría L.E., Dutra W.O., Gascon J., Morillo C.A., Oliveira-Filho J., et al. Chagas Cardiomyopathy: An Update of Current Clinical Knowledge and Management: A Scientific Statement From the American Heart Association. Circulation. 2018;138:e169–e209. doi: 10.1161/CIR.0000000000000599. - DOI - PubMed
LinkOut - more resources
Full Text Sources
Research Materials