The interplay between autophagy and cGAS-STING signaling and its implications for cancer
- PMID: 38660307
- PMCID: PMC11039819
- DOI: 10.3389/fimmu.2024.1356369
The interplay between autophagy and cGAS-STING signaling and its implications for cancer
Abstract
Autophagy is an intracellular process that targets various cargos for degradation, including members of the cGAS-STING signaling cascade. cGAS-STING senses cytosolic double-stranded DNA and triggers an innate immune response through type I interferons. Emerging evidence suggests that autophagy plays a crucial role in regulating and fine-tuning cGAS-STING signaling. Reciprocally, cGAS-STING pathway members can actively induce canonical as well as various non-canonical forms of autophagy, establishing a regulatory network of feedback mechanisms that alter both the cGAS-STING and the autophagic pathway. The crosstalk between autophagy and the cGAS-STING pathway impacts a wide variety of cellular processes such as protection against pathogenic infections as well as signaling in neurodegenerative disease, autoinflammatory disease and cancer. Here we provide a comprehensive overview of the mechanisms involved in autophagy and cGAS-STING signaling, with a specific focus on the interactions between the two pathways and their importance for cancer.
Keywords: autophagy; cGAS/STING signaling; cancer; innate immunity; radiotherapy.
Copyright © 2024 Schmid, Fischer, Engl, Widder, Kerschbaum-Gruber and Slade.
Conflict of interest statement
The authors declare that the research was conducted in the absence of any commercial or financial relationships that could be construed as a potential conflict of interest.
Figures
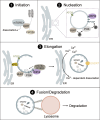
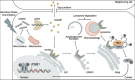
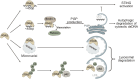
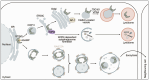
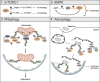
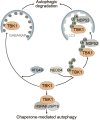
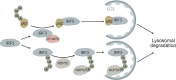
Similar articles
-
How the Innate Immune DNA Sensing cGAS-STING Pathway Is Involved in Autophagy.Int J Mol Sci. 2021 Dec 8;22(24):13232. doi: 10.3390/ijms222413232. Int J Mol Sci. 2021. PMID: 34948027 Free PMC article. Review.
-
Crosstalk between cGAS-STING pathway and autophagy in cancer immunity.Front Immunol. 2023 Mar 1;14:1139595. doi: 10.3389/fimmu.2023.1139595. eCollection 2023. Front Immunol. 2023. PMID: 36936940 Free PMC article. Review.
-
The cGAS-STING-autophagy pathway: Novel perspectives in neurotoxicity induced by manganese exposure.Environ Pollut. 2022 Dec 15;315:120412. doi: 10.1016/j.envpol.2022.120412. Epub 2022 Oct 12. Environ Pollut. 2022. PMID: 36240967 Review.
-
How the Innate Immune DNA Sensing cGAS-STING Pathway Is Involved in Apoptosis.Int J Mol Sci. 2023 Feb 3;24(3):3029. doi: 10.3390/ijms24033029. Int J Mol Sci. 2023. PMID: 36769349 Free PMC article. Review.
-
Targeting protein condensation in cGAS-STING signaling pathway.Bioessays. 2024 Sep;46(9):e2400091. doi: 10.1002/bies.202400091. Epub 2024 Jul 4. Bioessays. 2024. PMID: 38962845 Review.
Cited by
-
Autophagy and autophagic cell death in sepsis: friend or foe?J Intensive Care. 2024 Oct 25;12(1):41. doi: 10.1186/s40560-024-00754-y. J Intensive Care. 2024. PMID: 39449054 Free PMC article. Review.
-
Clinical applications of STING agonists in cancer immunotherapy: current progress and future prospects.Front Immunol. 2024 Oct 2;15:1485546. doi: 10.3389/fimmu.2024.1485546. eCollection 2024. Front Immunol. 2024. PMID: 39421752 Free PMC article. Review.
-
Recent advancements in cGAS-STING activation, tumor immune evasion, and therapeutic implications.Med Oncol. 2024 Oct 18;41(11):291. doi: 10.1007/s12032-024-02539-7. Med Oncol. 2024. PMID: 39419913 Review.
References
Publication types
MeSH terms
Substances
Grants and funding
LinkOut - more resources
Full Text Sources
Research Materials