Adenylyl cyclase isoforms 5 and 6 in the cardiovascular system: complex regulation and divergent roles
- PMID: 38633617
- PMCID: PMC11021717
- DOI: 10.3389/fphar.2024.1370506
Adenylyl cyclase isoforms 5 and 6 in the cardiovascular system: complex regulation and divergent roles
Abstract
Adenylyl cyclases (ACs) are crucial effector enzymes that transduce divergent signals from upstream receptor pathways and are responsible for catalyzing the conversion of ATP to cAMP. The ten AC isoforms are categorized into four main groups; the class III or calcium-inhibited family of ACs comprises AC5 and AC6. These enzymes are very closely related in structure and have a paucity of selective activators or inhibitors, making it difficult to distinguish them experimentally. AC5 and AC6 are highly expressed in the heart and vasculature, as well as the spinal cord and brain; AC6 is also abundant in the lungs, kidney, and liver. However, while AC5 and AC6 have similar expression patterns with some redundant functions, they have distinct physiological roles due to differing regulation and cAMP signaling compartmentation. AC5 is critical in cardiac and vascular function; AC6 is a key effector of vasodilatory pathways in vascular myocytes and is enriched in fetal/neonatal tissues. Expression of both AC5 and AC6 decreases in heart failure; however, AC5 disruption is cardio-protective, while overexpression of AC6 rescues cardiac function in cardiac injury. This is a comprehensive review of the complex regulation of AC5 and AC6 in the cardiovascular system, highlighting overexpression and knockout studies as well as transgenic models illuminating each enzyme and focusing on post-translational modifications that regulate their cellular localization and biological functions. We also describe pharmacological challenges in the design of isoform-selective activators or inhibitors for AC5 and AC6, which may be relevant to developing new therapeutic approaches for several cardiovascular diseases.
Keywords: G protein-coupled receptors; adenylyl cyclase; cyclic 3′,5′-adenosine monophosphate; drug discovery; heart disease; signal transduction.
Copyright © 2024 Maghsoudi, Shuaib, Van Bastelaere and Dakshinamurti.
Conflict of interest statement
The authors declare that the research was conducted in the absence of any commercial or financial relationships that could be construed as a potential conflict of interest. The author(s) declared that they were an editorial board member of Frontiers, at the time of submission. This had no impact on the peer review process and the final decision.
Figures
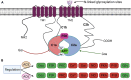
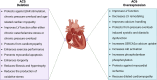
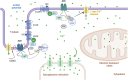
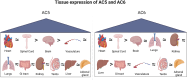
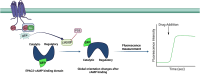
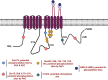





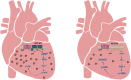
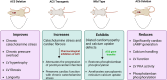
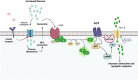
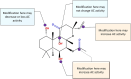
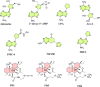
Similar articles
-
Qualitative evidence synthesis informing our understanding of people's perceptions and experiences of targeted digital communication.Cochrane Database Syst Rev. 2019 Oct 23;10(10):ED000141. doi: 10.1002/14651858.ED000141. Cochrane Database Syst Rev. 2019. PMID: 31643081 Free PMC article.
-
Depressing time: Waiting, melancholia, and the psychoanalytic practice of care.In: Kirtsoglou E, Simpson B, editors. The Time of Anthropology: Studies of Contemporary Chronopolitics. Abingdon: Routledge; 2020. Chapter 5. In: Kirtsoglou E, Simpson B, editors. The Time of Anthropology: Studies of Contemporary Chronopolitics. Abingdon: Routledge; 2020. Chapter 5. PMID: 36137063 Free Books & Documents. Review.
-
Using Experience Sampling Methodology to Capture Disclosure Opportunities for Autistic Adults.Autism Adulthood. 2023 Dec 1;5(4):389-400. doi: 10.1089/aut.2022.0090. Epub 2023 Dec 12. Autism Adulthood. 2023. PMID: 38116059 Free PMC article.
-
Radiation protection following nuclear power accidents: a survey of putative mechanisms involved in the radioprotective actions of taurine during and after radiation exposure.Microb Ecol Health Dis. 2012 Feb 1;23. doi: 10.3402/mehd.v23i0.14787. eCollection 2012. Microb Ecol Health Dis. 2012. PMID: 23990836 Free PMC article.
-
Pharmacological treatments in panic disorder in adults: a network meta-analysis.Cochrane Database Syst Rev. 2023 Nov 28;11(11):CD012729. doi: 10.1002/14651858.CD012729.pub3. Cochrane Database Syst Rev. 2023. PMID: 38014714 Free PMC article. Review.
References
-
- Bai Y., Tsunematsu T., Jiao Q., Ohnuki Y., Mototani Y., Shiozawa K., et al. (2012). Pharmacological stimulation of type 5 adenylyl cyclase stabilizes heart rate under both microgravity and hypergravity induced by parabolic flight. J. Pharmacol. Sci. 119 (4), 381–389. 10.1254/jphs.12102fp - DOI - PubMed
Publication types
Grants and funding
LinkOut - more resources
Full Text Sources