Thermal escape box: A cost-benefit evaluation paradigm for investigating thermosensation and thermal pain
- PMID: 38617105
- PMCID: PMC11015515
- DOI: 10.1016/j.ynpai.2024.100155
Thermal escape box: A cost-benefit evaluation paradigm for investigating thermosensation and thermal pain
Abstract
Thermosensation, the ability to detect and estimate temperature, is an evolutionarily conserved process that is essential for survival. Thermosensing is impaired in various pain syndromes, resulting in thermal allodynia, the perception of an innocuous temperature as painful, or thermal hyperalgesia, an exacerbated perception of a painful thermal stimulus. Several behavioral assays exist to study thermosensation and thermal pain in rodents, however, most rely on reflexive withdrawal responses or the subjective quantification of spontaneous nocifensive behaviors. Here, we created a new apparatus, the thermal escape box, which can be attached to temperature-controlled plates and used to assess temperature-dependent effort-based decision-making. The apparatus consists of a light chamber with an opening that fits around temperature-controlled plates, and a small entryway into a dark chamber. A mouse must choose to stay in a brightly lit aversive area or traverse the plates to escape to the enclosed dark chamber. We quantified escape latencies of adult C57Bl/6 mice at different plate temperatures from video recordings and found they were significantly longer at 5 °C, 18 °C, and 52 °C, compared to 30 °C, a mouse's preferred ambient temperature. Differences in escape latencies were abolished in male Trpm8-/- mice and in male Trpv1-/- animals. Finally, we show that chronic constriction injury procedures or oxaliplatin treatement significantly increased escape latencies at cold temperatures compared to controls, the later of which was prevented by the analgesic meloxicam. This demonstrates the utility of this assay in detecting cold pain. Collectively, our study has identified a new and effective tool that uses cost-benefit valuations to study thermosensation and thermal pain.
Keywords: Cost-benefit analysis; Decision-based behavior; Nociception; Thermal allodynia; Thermal hyperalgesia; Thermosensation.
© 2024 The Authors. Published by Elsevier Inc.
Conflict of interest statement
The authors declare the following financial interests/personal relationships which may be considered as potential competing interests: Theanne N. Griffith has assigned a provisional patent to the University of California related to the described behavioral assay. Other authors report no conflicts of interest.
Figures
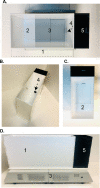
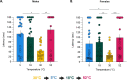
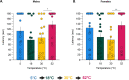
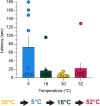
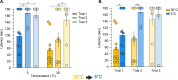
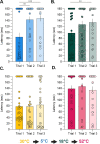
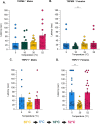
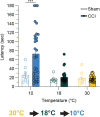
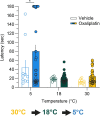
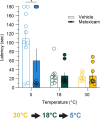
Similar articles
-
Depressing time: Waiting, melancholia, and the psychoanalytic practice of care.In: Kirtsoglou E, Simpson B, editors. The Time of Anthropology: Studies of Contemporary Chronopolitics. Abingdon: Routledge; 2020. Chapter 5. In: Kirtsoglou E, Simpson B, editors. The Time of Anthropology: Studies of Contemporary Chronopolitics. Abingdon: Routledge; 2020. Chapter 5. PMID: 36137063 Free Books & Documents. Review.
-
Defining the optimum strategy for identifying adults and children with coeliac disease: systematic review and economic modelling.Health Technol Assess. 2022 Oct;26(44):1-310. doi: 10.3310/ZUCE8371. Health Technol Assess. 2022. PMID: 36321689 Free PMC article.
-
Far Posterior Approach for Rib Fracture Fixation: Surgical Technique and Tips.JBJS Essent Surg Tech. 2024 Dec 6;14(4):e23.00094. doi: 10.2106/JBJS.ST.23.00094. eCollection 2024 Oct-Dec. JBJS Essent Surg Tech. 2024. PMID: 39650795 Free PMC article.
-
Trends in Surgical and Nonsurgical Aesthetic Procedures: A 14-Year Analysis of the International Society of Aesthetic Plastic Surgery-ISAPS.Aesthetic Plast Surg. 2024 Oct;48(20):4217-4227. doi: 10.1007/s00266-024-04260-2. Epub 2024 Aug 5. Aesthetic Plast Surg. 2024. PMID: 39103642 Review.
-
Can a Liquid Biopsy Detect Circulating Tumor DNA With Low-passage Whole-genome Sequencing in Patients With a Sarcoma? A Pilot Evaluation.Clin Orthop Relat Res. 2025 Jan 1;483(1):39-48. doi: 10.1097/CORR.0000000000003161. Epub 2024 Jun 21. Clin Orthop Relat Res. 2025. PMID: 38905450
References
-
- Braden K., Stratton H.J., Salvemini D., Khanna R. Small molecule targeting NaV1.7 via inhibition of the CRMP2-Ubc9 interaction reduces and prevents pain chronification in a mouse model of oxaliplatin-induced neuropathic pain. neurobiol. Pain. 2022;11 doi: 10.1016/j.ynpai.2021.100082. - DOI - PMC - PubMed