Camel-Derived Nanobodies as Potent Inhibitors of New Delhi Metallo-β-Lactamase-1 Enzyme
- PMID: 38611711
- PMCID: PMC11013165
- DOI: 10.3390/molecules29071431
Camel-Derived Nanobodies as Potent Inhibitors of New Delhi Metallo-β-Lactamase-1 Enzyme
Abstract
The injudicious usage of antibiotics during infections caused by Gram-negative bacteria leads to the emergence of β-lactamases. Among them, the NDM-1 enzyme poses a serious threat to human health. Developing new antibiotics or inhibiting β-lactamases might become essential to reduce and prevent bacterial infections. Nanobodies (Nbs), the smallest antigen-binding single-domain fragments derived from Camelidae heavy-chain-only antibodies, targeting enzymes, are innovative alternatives to develop effective inhibitors. The biopanning of an immune VHH library after phage display has helped to retrieve recombinant antibody fragments with high inhibitory activity against recombinant-NDM-1 enzyme. Nb02NDM-1, Nb12NDM-1, and Nb17NDM-1 behaved as uncompetitive inhibitors against NDM-1 with Ki values in the nM range. Remarkably, IC50 values of 25.0 nM and 8.5 nM were noted for Nb02NDM-1 and Nb17NDM-1, respectively. The promising inhibition of NDM-1 by Nbs highlights their potential application in combating particular Gram-negative infections.
Keywords: NDM-1; metallo-β-lactamases; nanobodies; phage display; β-lactamase small molecule inhibitors.
Conflict of interest statement
The authors declare not conflict of interest.
Figures
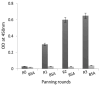
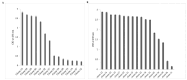
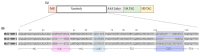
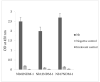
Similar articles
-
Inhibitory Potential of Polyclonal Camel Antibodies against New Delhi Metallo-β-lactamase-1 (NDM-1).Molecules. 2020 Sep 28;25(19):4453. doi: 10.3390/molecules25194453. Molecules. 2020. PMID: 32998307 Free PMC article.
-
Stereochemically altered cephalosporins as potent inhibitors of New Delhi metallo-β-lactamases.Eur J Med Chem. 2022 Mar 15;232:114174. doi: 10.1016/j.ejmech.2022.114174. Epub 2022 Feb 4. Eur J Med Chem. 2022. PMID: 35152091
-
Novel metallo-β-lactamases inhibitors restore the susceptibility of carbapenems to New Delhi metallo-lactamase-1 (NDM-1)-harbouring bacteria.Br J Pharmacol. 2024 Jan;181(1):54-69. doi: 10.1111/bph.16210. Epub 2023 Sep 6. Br J Pharmacol. 2024. PMID: 37539785
-
Potential Inhibitors Against NDM-1 Type Metallo-β-Lactamases: An Overview.Microb Drug Resist. 2020 Dec;26(12):1568-1588. doi: 10.1089/mdr.2019.0315. Epub 2020 Jun 2. Microb Drug Resist. 2020. PMID: 32486911 Review.
-
Efforts towards the inhibitor design for New Delhi metallo-beta-lactamase (NDM-1).Eur J Med Chem. 2021 Dec 5;225:113747. doi: 10.1016/j.ejmech.2021.113747. Epub 2021 Aug 5. Eur J Med Chem. 2021. PMID: 34391033 Review.
References
-
- Solomon S.L., Oliver K.B. Antibiotic resistance threats in the United States: Stepping back from the brink. Am. Fam. Physician. 2014;89:938–941. - PubMed
MeSH terms
Substances
Grants and funding
LinkOut - more resources
Full Text Sources
Research Materials
Miscellaneous