Endogenous trans-translation structure visualizes the decoding of the first tmRNA alanine codon
- PMID: 38500588
- PMCID: PMC10944890
- DOI: 10.3389/fmicb.2024.1369760
Endogenous trans-translation structure visualizes the decoding of the first tmRNA alanine codon
Abstract
Ribosomes stall on truncated or otherwise damaged mRNAs. Bacteria rely on ribosome rescue mechanisms to replenish the pool of ribosomes available for translation. Trans-translation, the main ribosome-rescue pathway, uses a circular hybrid transfer-messenger RNA (tmRNA) to restart translation and label the resulting peptide for degradation. Previous studies have visualized how tmRNA and its helper protein SmpB interact with the stalled ribosome to establish a new open reading frame. As tmRNA presents the first alanine codon via a non-canonical mRNA path in the ribosome, the incoming alanyl-tRNA must rearrange the tmRNA molecule to read the codon. Here, we describe cryo-EM analyses of an endogenous Escherichia coli ribosome-tmRNA complex with tRNAAla accommodated in the A site. The flexible adenosine-rich tmRNA linker, which connects the mRNA-like domain with the codon, is stabilized by the minor groove of the canonically positioned anticodon stem of tRNAAla. This ribosome complex can also accommodate a tRNA near the E (exit) site, bringing insights into the translocation and dissociation of the tRNA that decoded the defective mRNA prior to tmRNA binding. Together, these structures uncover a key step of ribosome rescue, in which the ribosome starts translating the tmRNA reading frame.
Keywords: A-minor interactions; SmpB; alanyl-tRNA; cryo-EM; endogenous tmRNA; tmRNA decoding.
Copyright © 2024 Teran, Zhang and Korostelev.
Conflict of interest statement
The authors declare that the research was conducted in the absence of any commercial or financial relationships that could be construed as a potential conflict of interest.
Figures
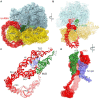
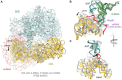
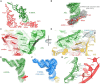
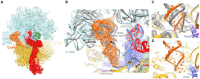
Similar articles
-
Depressing time: Waiting, melancholia, and the psychoanalytic practice of care.In: Kirtsoglou E, Simpson B, editors. The Time of Anthropology: Studies of Contemporary Chronopolitics. Abingdon: Routledge; 2020. Chapter 5. In: Kirtsoglou E, Simpson B, editors. The Time of Anthropology: Studies of Contemporary Chronopolitics. Abingdon: Routledge; 2020. Chapter 5. PMID: 36137063 Free Books & Documents. Review.
-
The ribosome-associated quality control pathway supports survival in the absence of non-stop ribosome rescue factors.mBio. 2024 Dec 11;15(12):e0232224. doi: 10.1128/mbio.02322-24. Epub 2024 Nov 13. mBio. 2024. PMID: 39535229 Free PMC article.
-
Far Posterior Approach for Rib Fracture Fixation: Surgical Technique and Tips.JBJS Essent Surg Tech. 2024 Dec 6;14(4):e23.00094. doi: 10.2106/JBJS.ST.23.00094. eCollection 2024 Oct-Dec. JBJS Essent Surg Tech. 2024. PMID: 39650795 Free PMC article.
-
RqcH supports survival in the absence of non-stop ribosome rescue factors.bioRxiv [Preprint]. 2024 Jul 12:2024.07.12.603306. doi: 10.1101/2024.07.12.603306. bioRxiv. 2024. Update in: mBio. 2024 Dec 11;15(12):e0232224. doi: 10.1128/mbio.02322-24. PMID: 39026760 Free PMC article. Updated. Preprint.
-
Genetic analysis of translation initiation in bacteria: An initiator tRNA-centric view.Mol Microbiol. 2024 Nov;122(5):772-788. doi: 10.1111/mmi.15243. Epub 2024 Feb 27. Mol Microbiol. 2024. PMID: 38410838 Review.
References
Grants and funding
LinkOut - more resources
Full Text Sources
Research Materials