The Chemical Reactivity of Membrane Lipids
- PMID: 38498932
- PMCID: PMC10979411
- DOI: 10.1021/acs.chemrev.3c00608
The Chemical Reactivity of Membrane Lipids
Abstract
It is well-known that aqueous dispersions of phospholipids spontaneously assemble into bilayer structures. These structures have numerous applications across chemistry and materials science and form the fundamental structural unit of the biological membrane. The particular environment of the lipid bilayer, with a water-poor low dielectric core surrounded by a more polar and better hydrated interfacial region, gives the membrane particular biophysical and physicochemical properties and presents a unique environment for chemical reactions to occur. Many different types of molecule spanning a range of sizes, from dissolved gases through small organics to proteins, are able to interact with membranes and promote chemical changes to lipids that subsequently affect the physicochemical properties of the bilayer. This Review describes the chemical reactivity exhibited by lipids in their membrane form, with an emphasis on conditions where the lipids are well hydrated in the form of bilayers. Key topics include the following: lytic reactions of glyceryl esters, including hydrolysis, aminolysis, and transesterification; oxidation reactions of alkenes in unsaturated fatty acids and sterols, including autoxidation and oxidation by singlet oxygen; reactivity of headgroups, particularly with reactive carbonyl species; and E/Z isomerization of alkenes. The consequences of reactivity for biological activity and biophysical properties are also discussed.
Conflict of interest statement
The authors declare no competing financial interest.
Figures
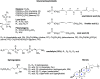
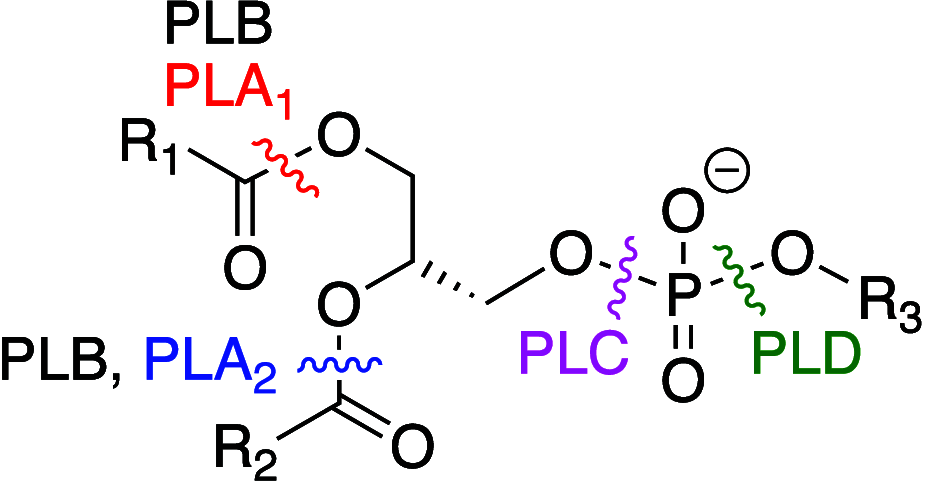
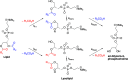
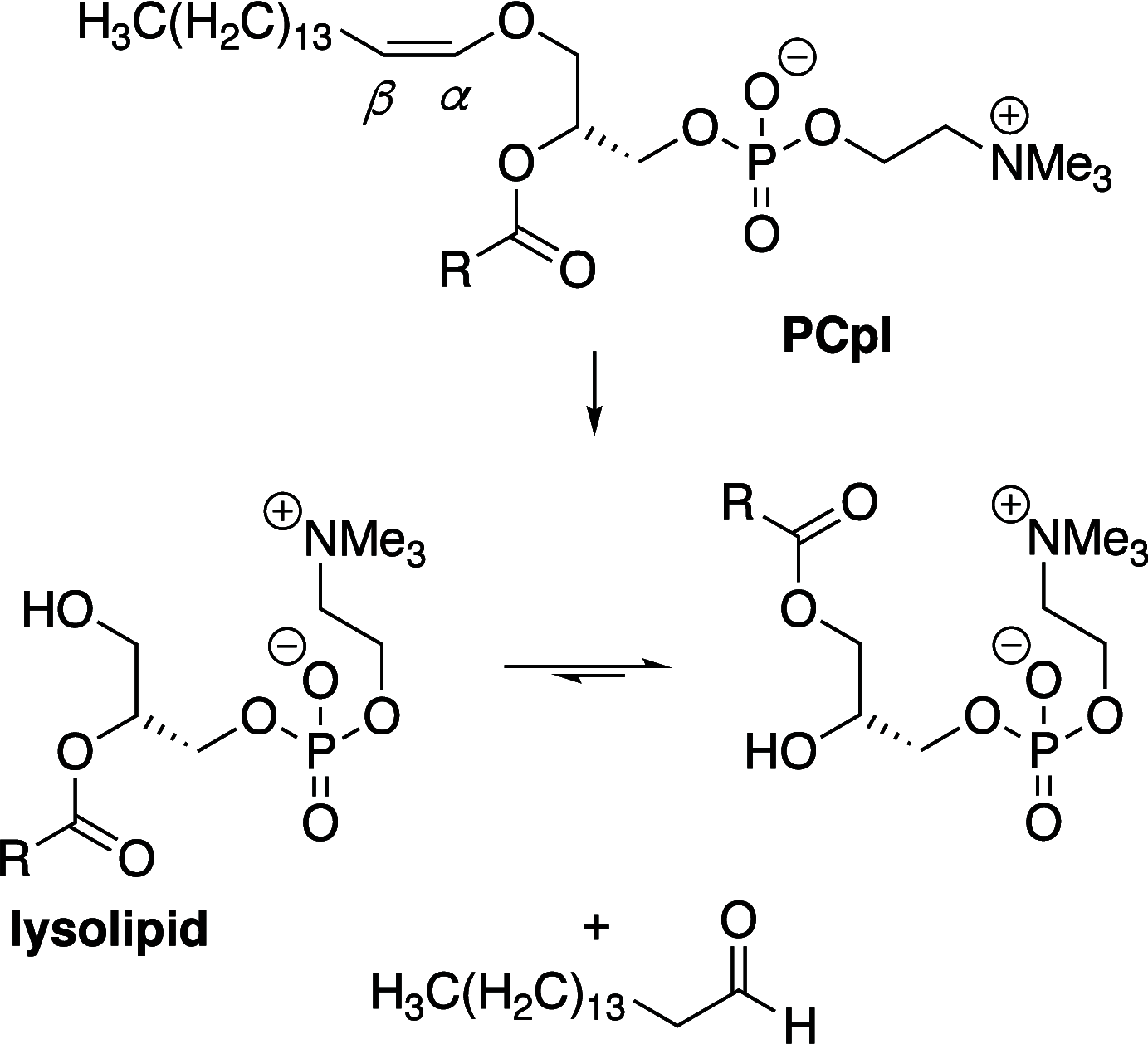
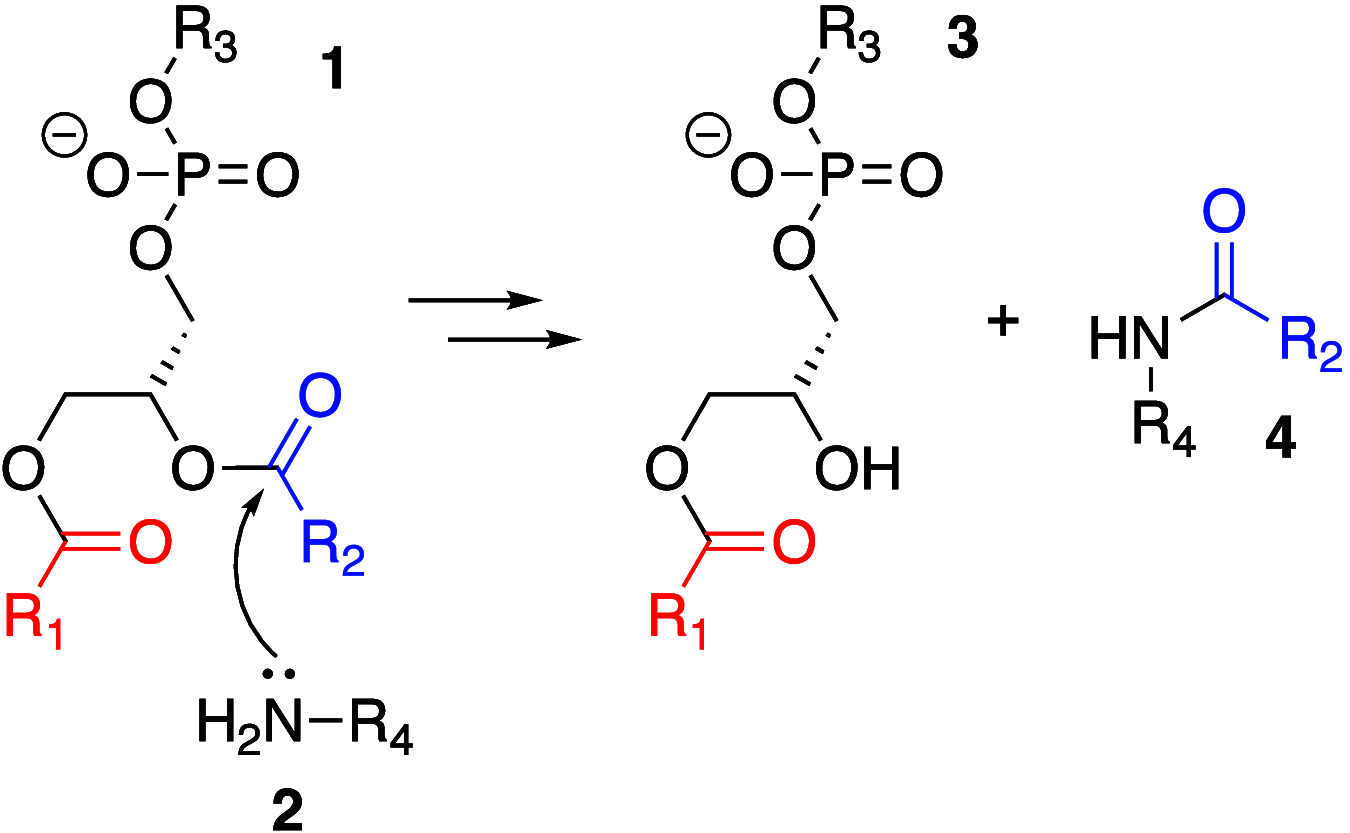
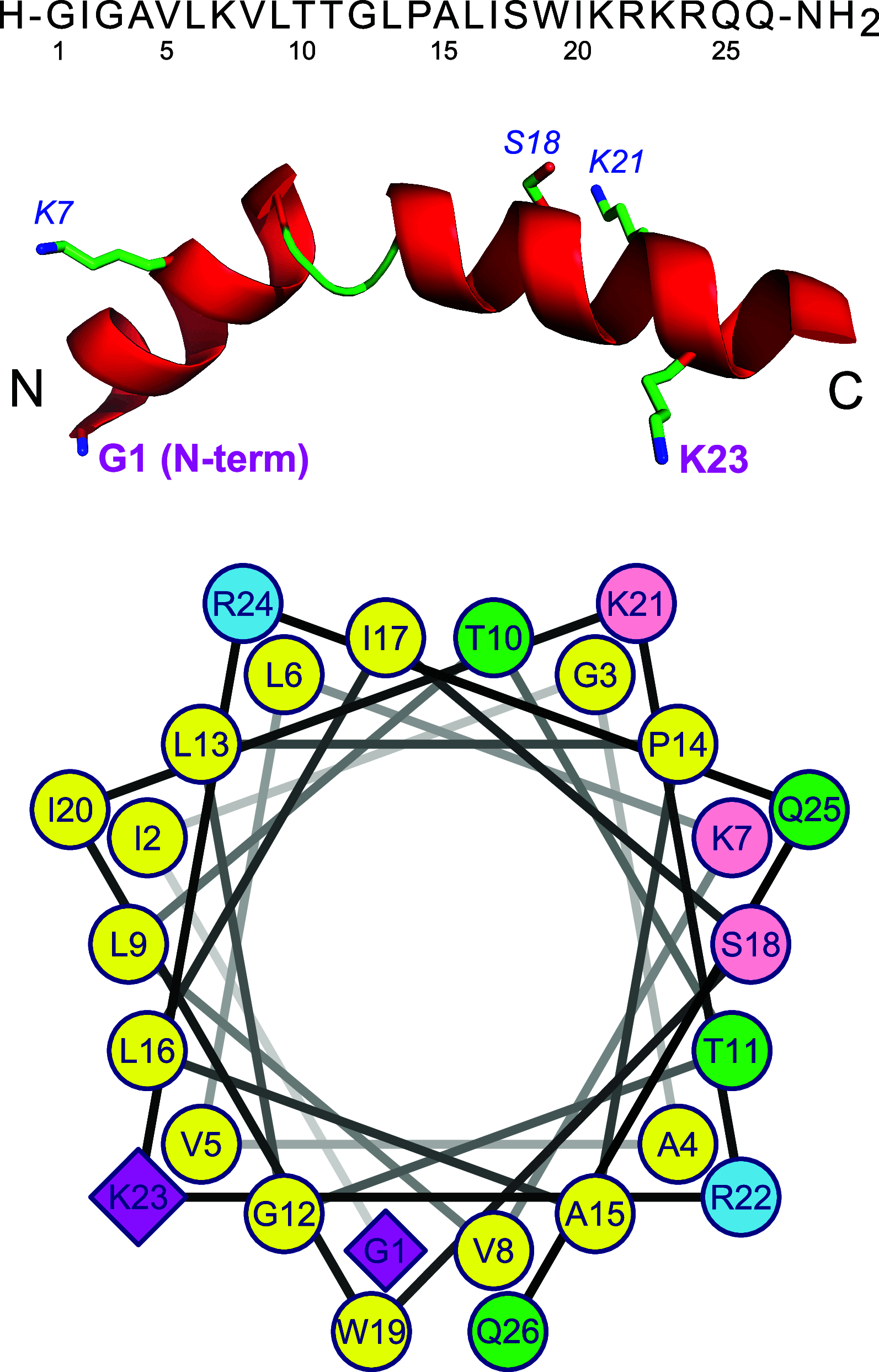
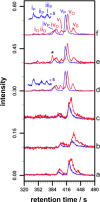
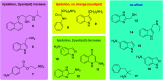
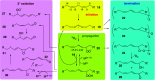
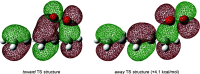
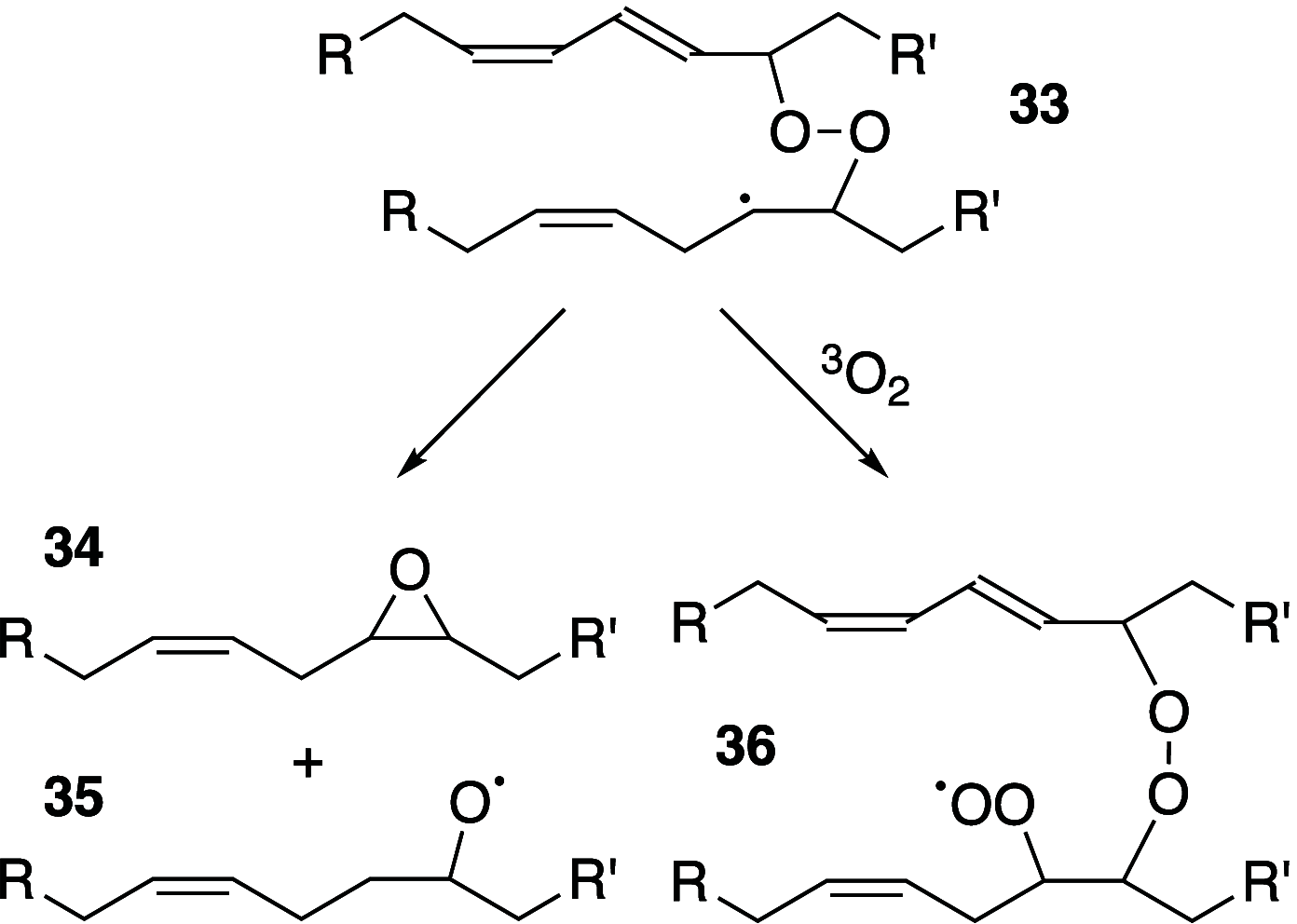
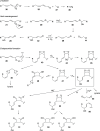
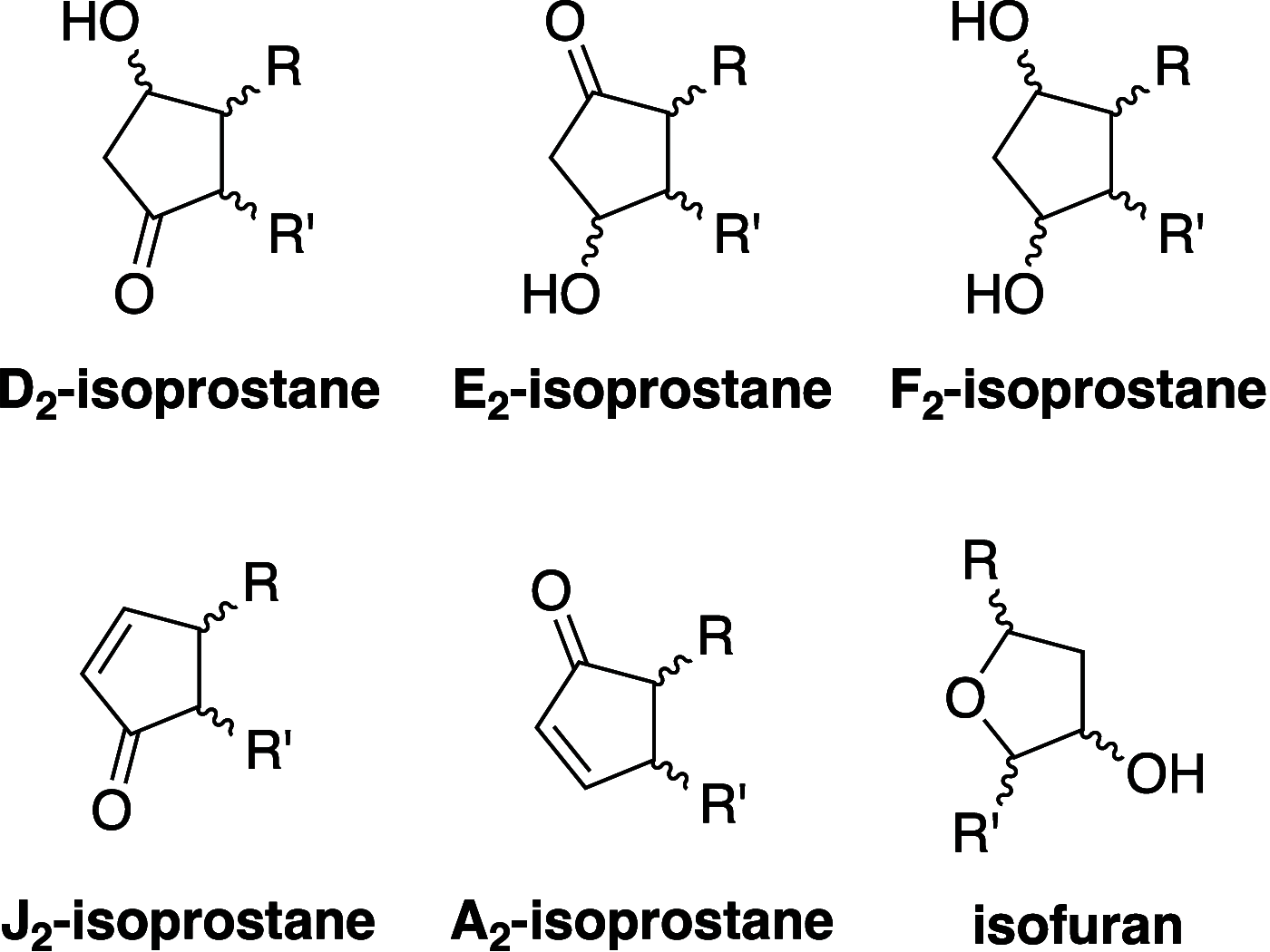
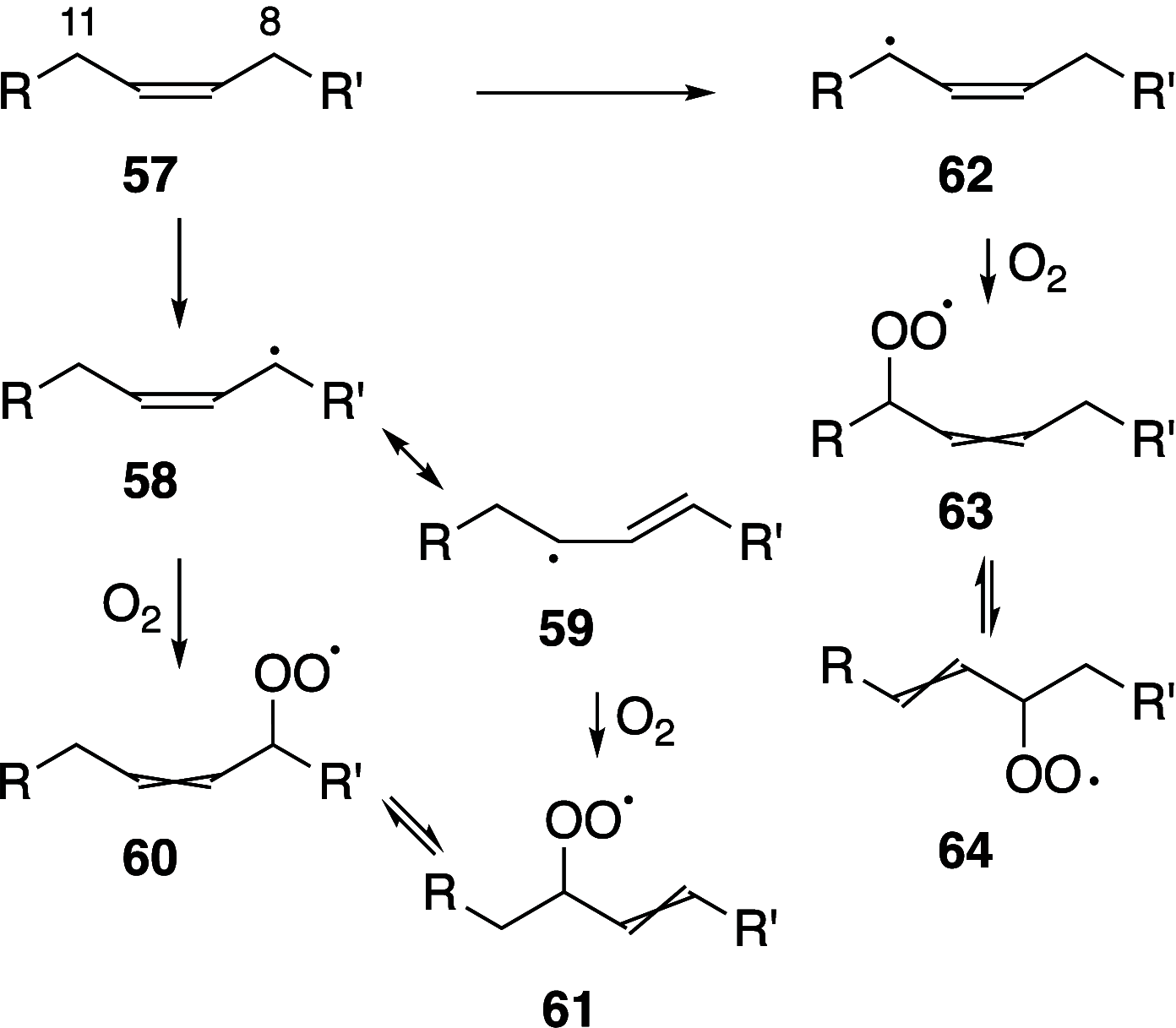
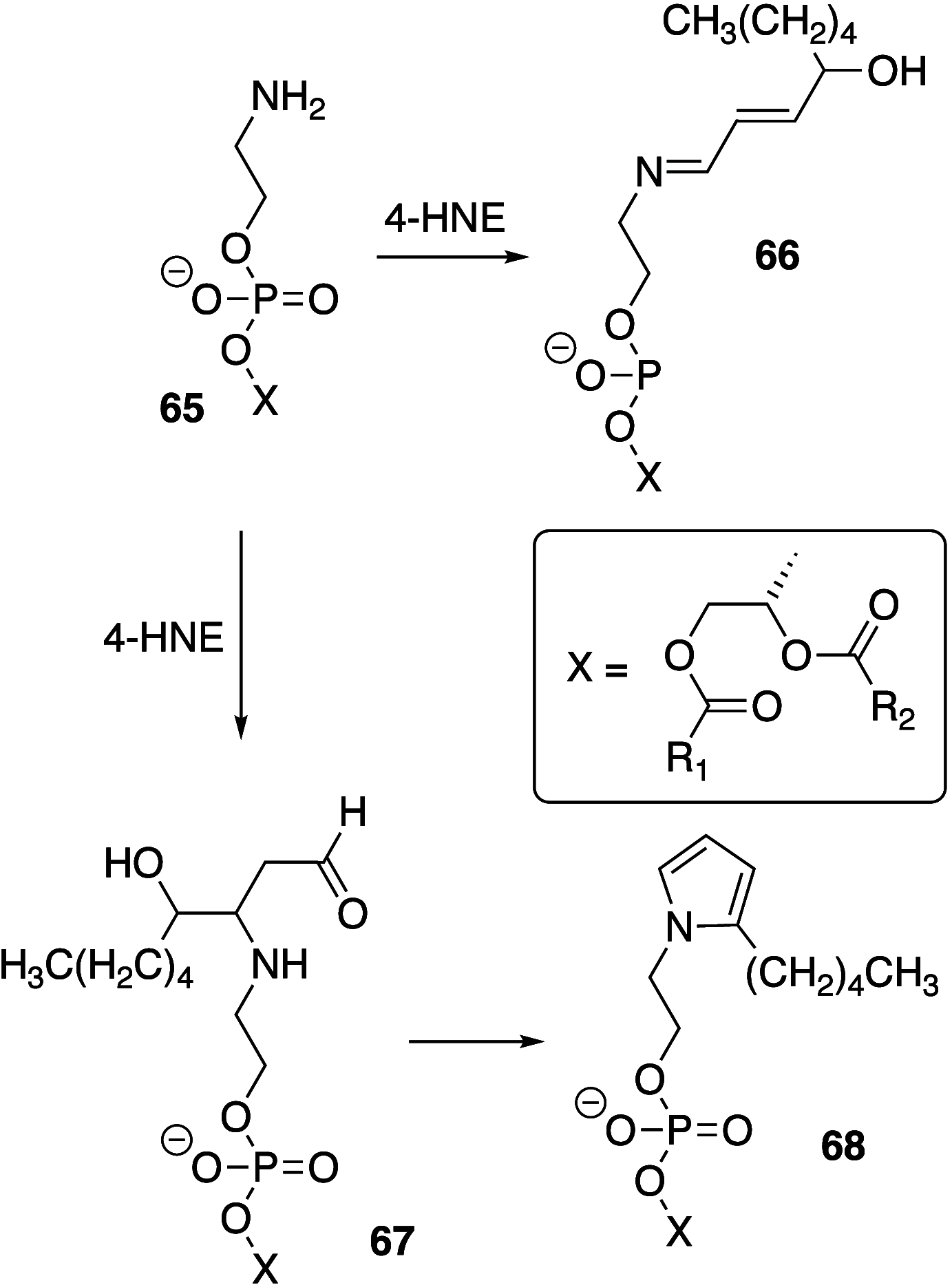
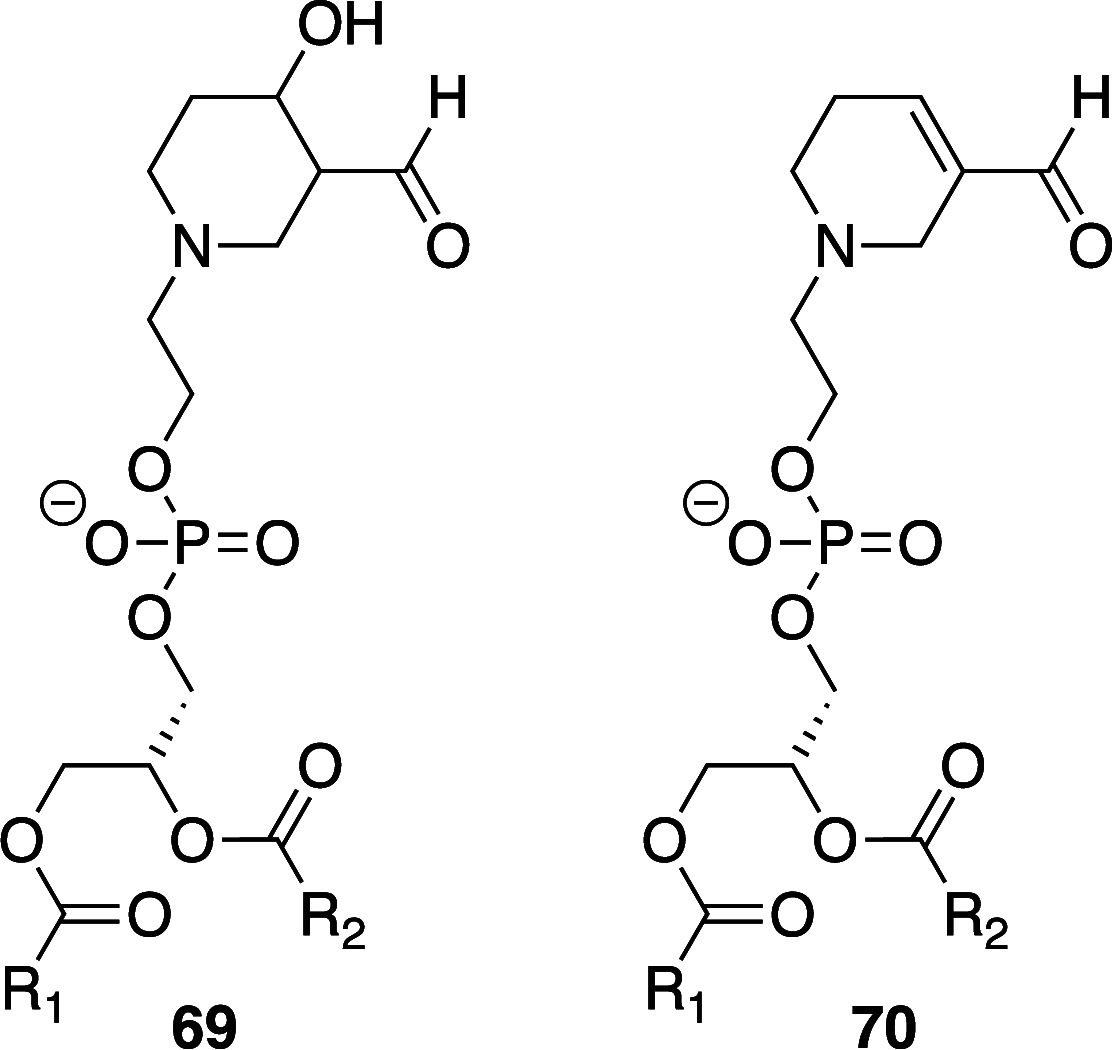
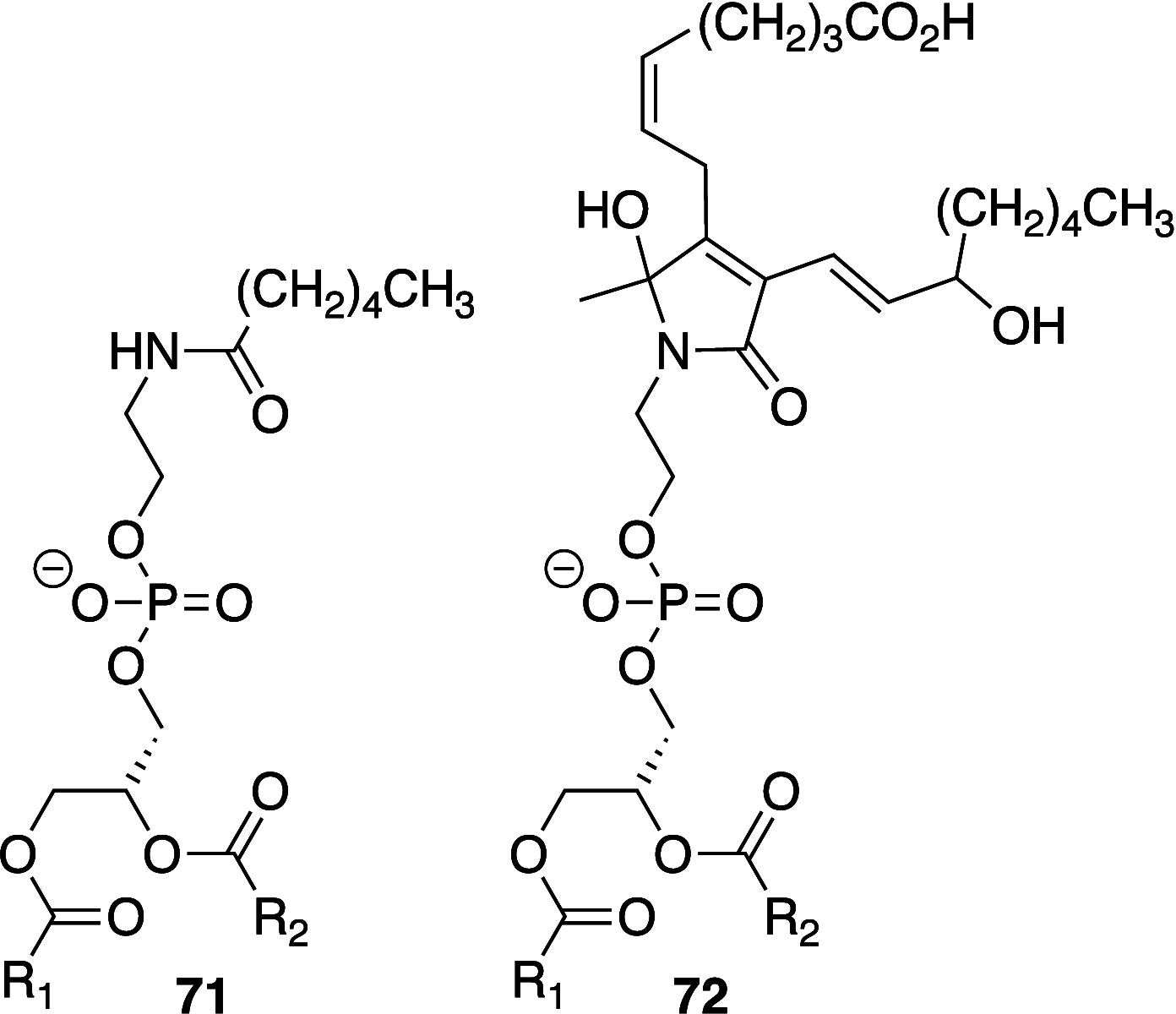
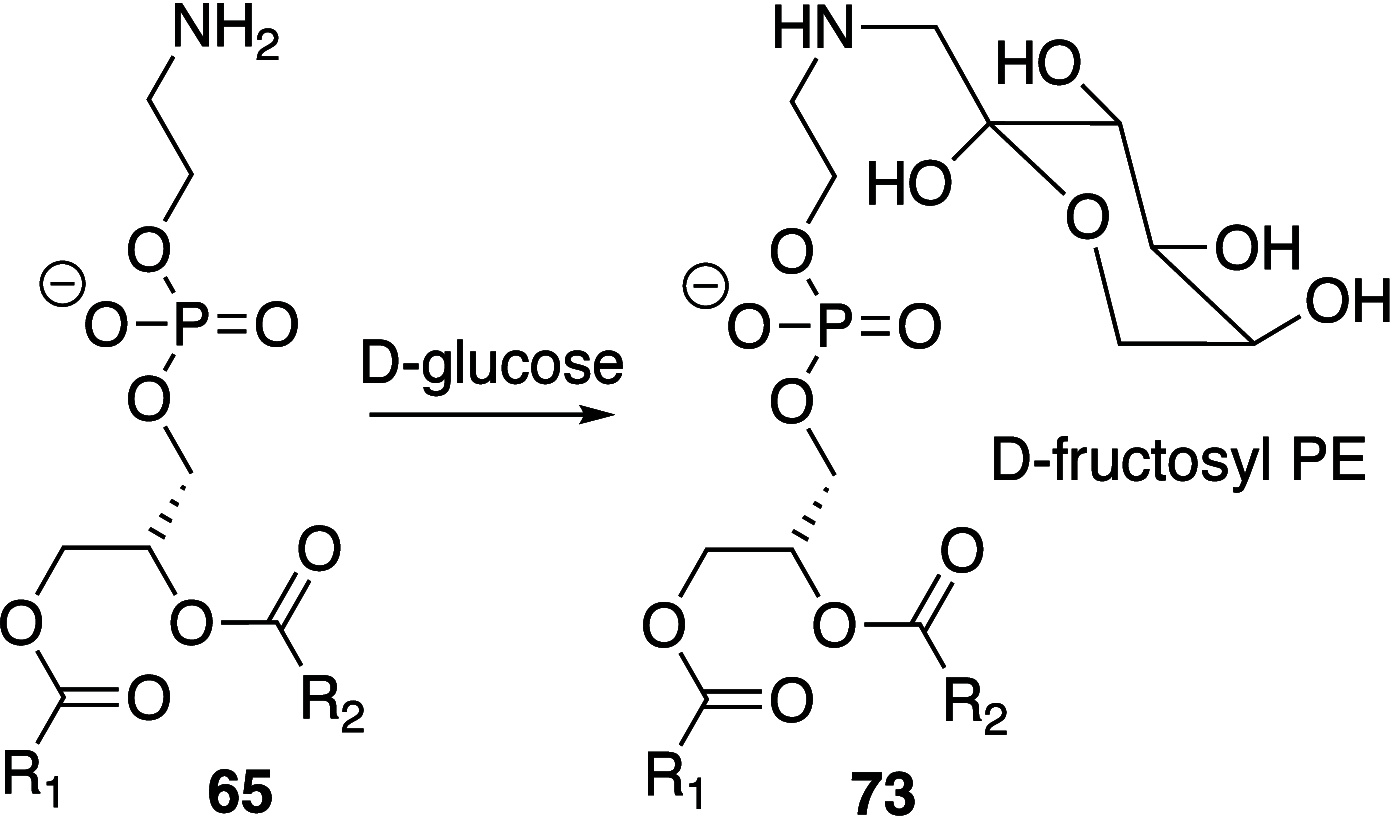
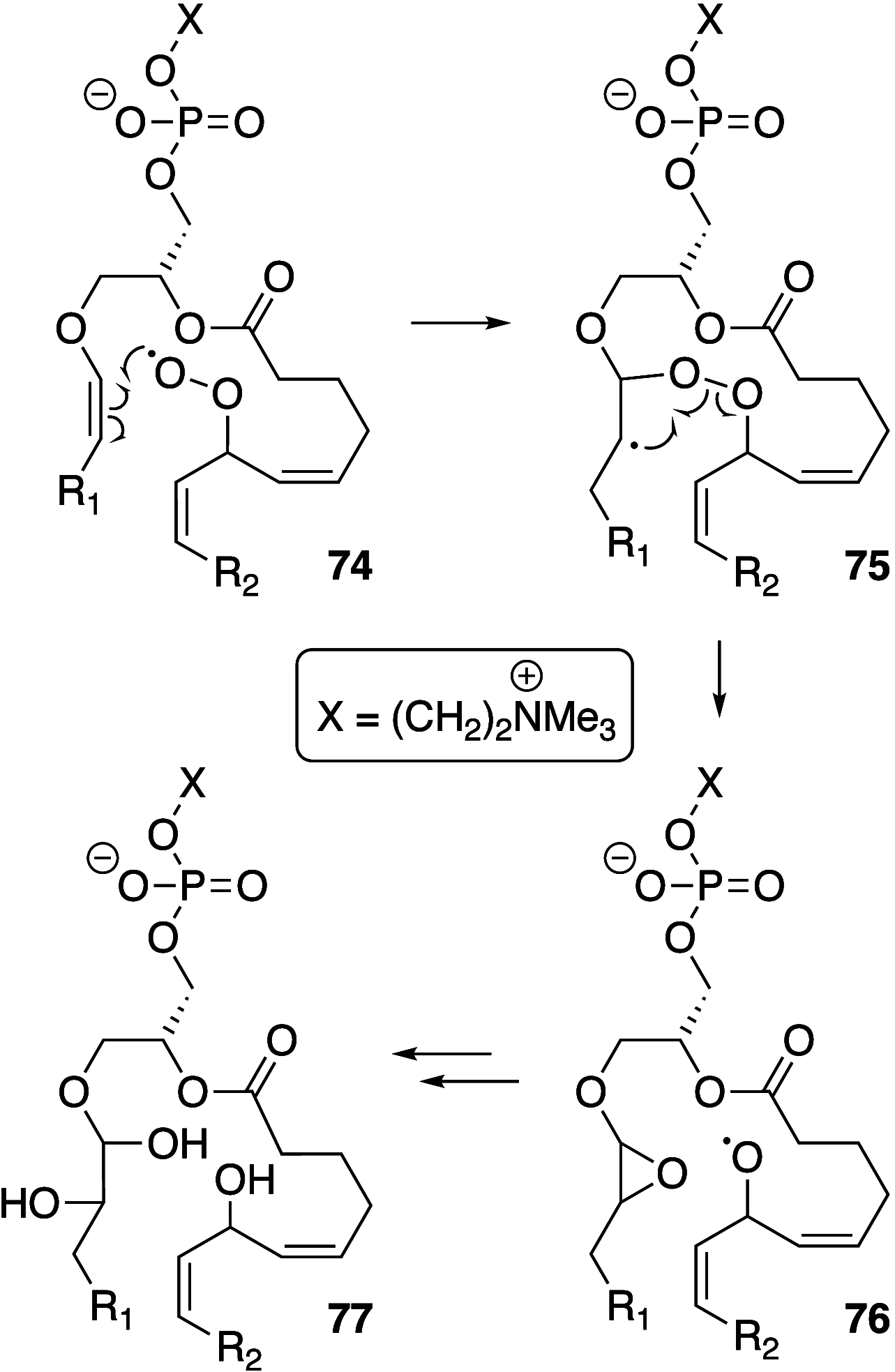
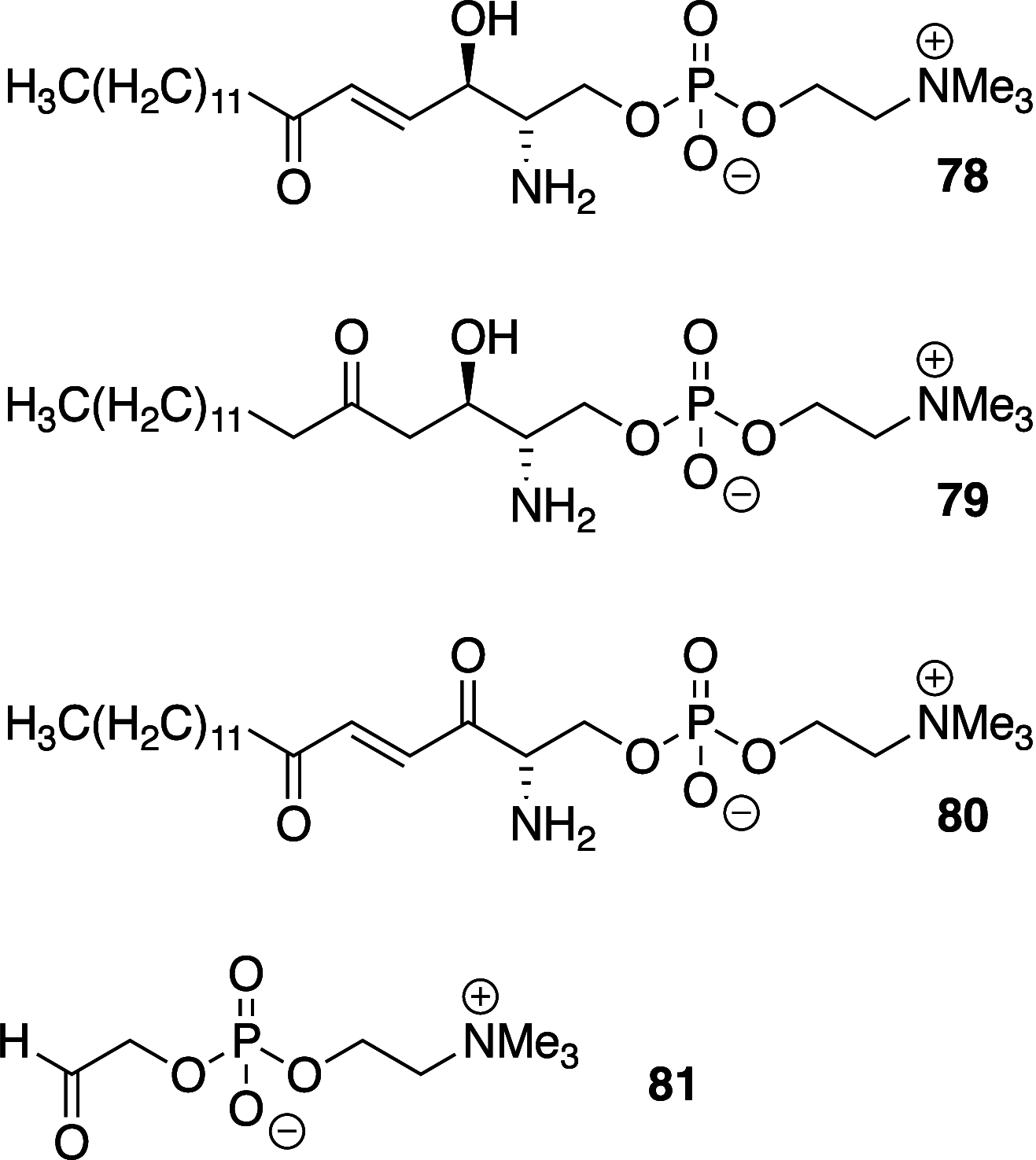
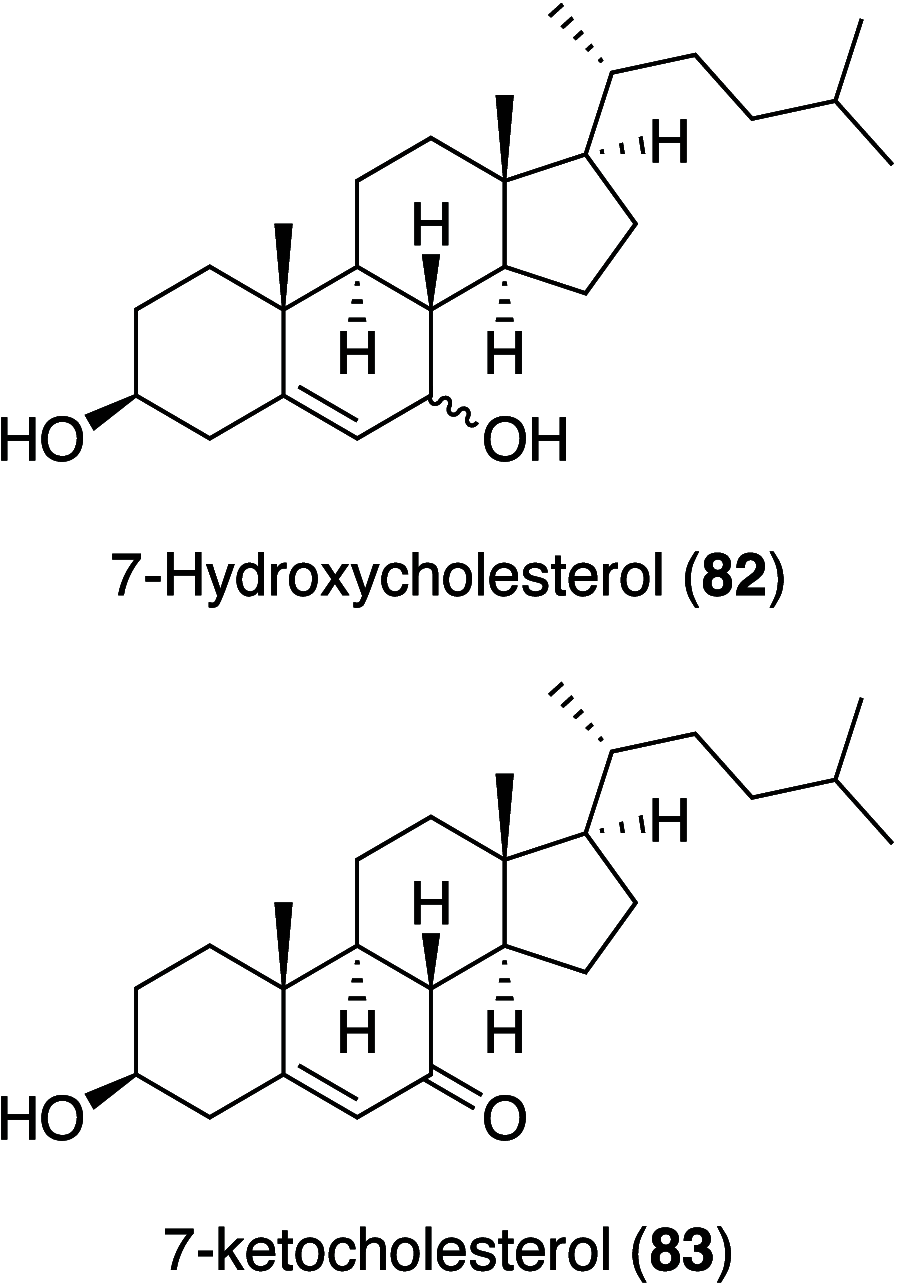
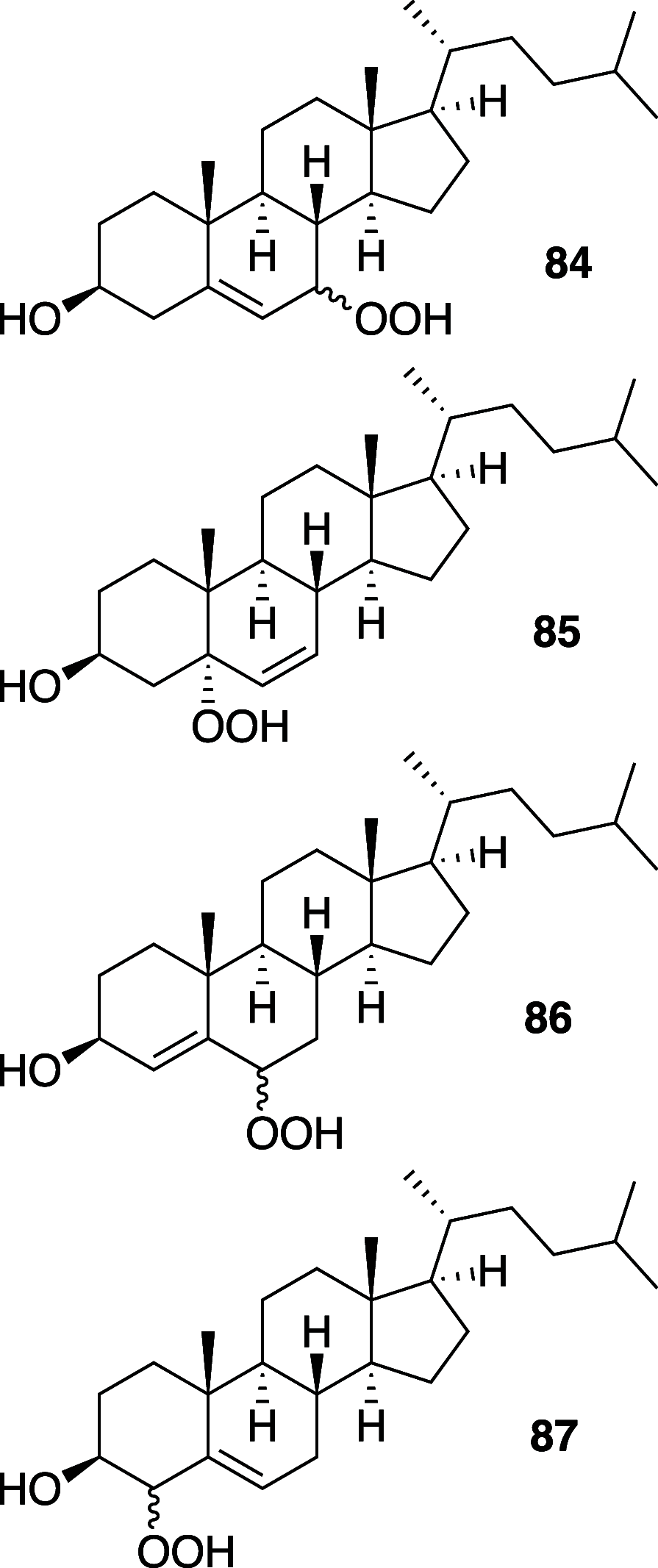
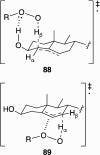
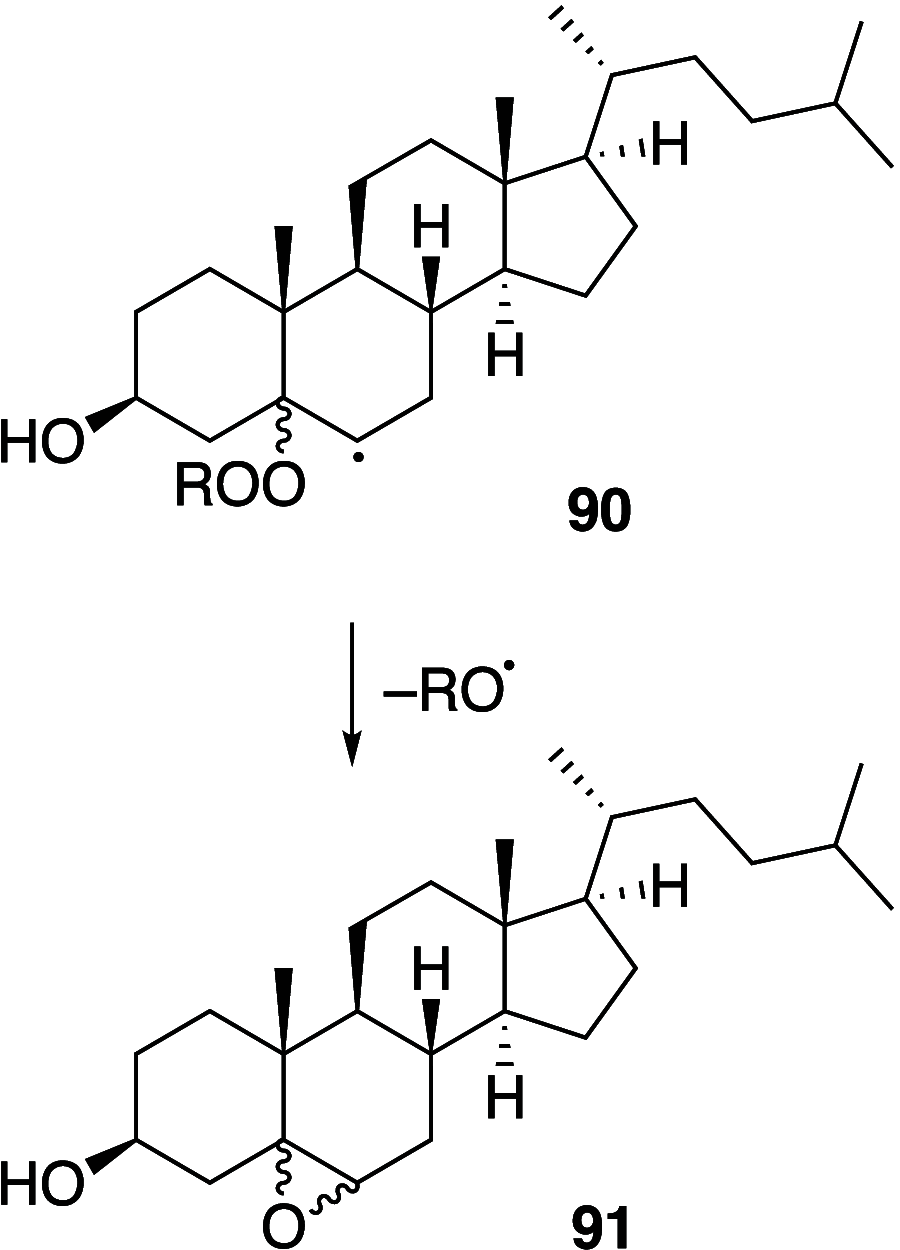
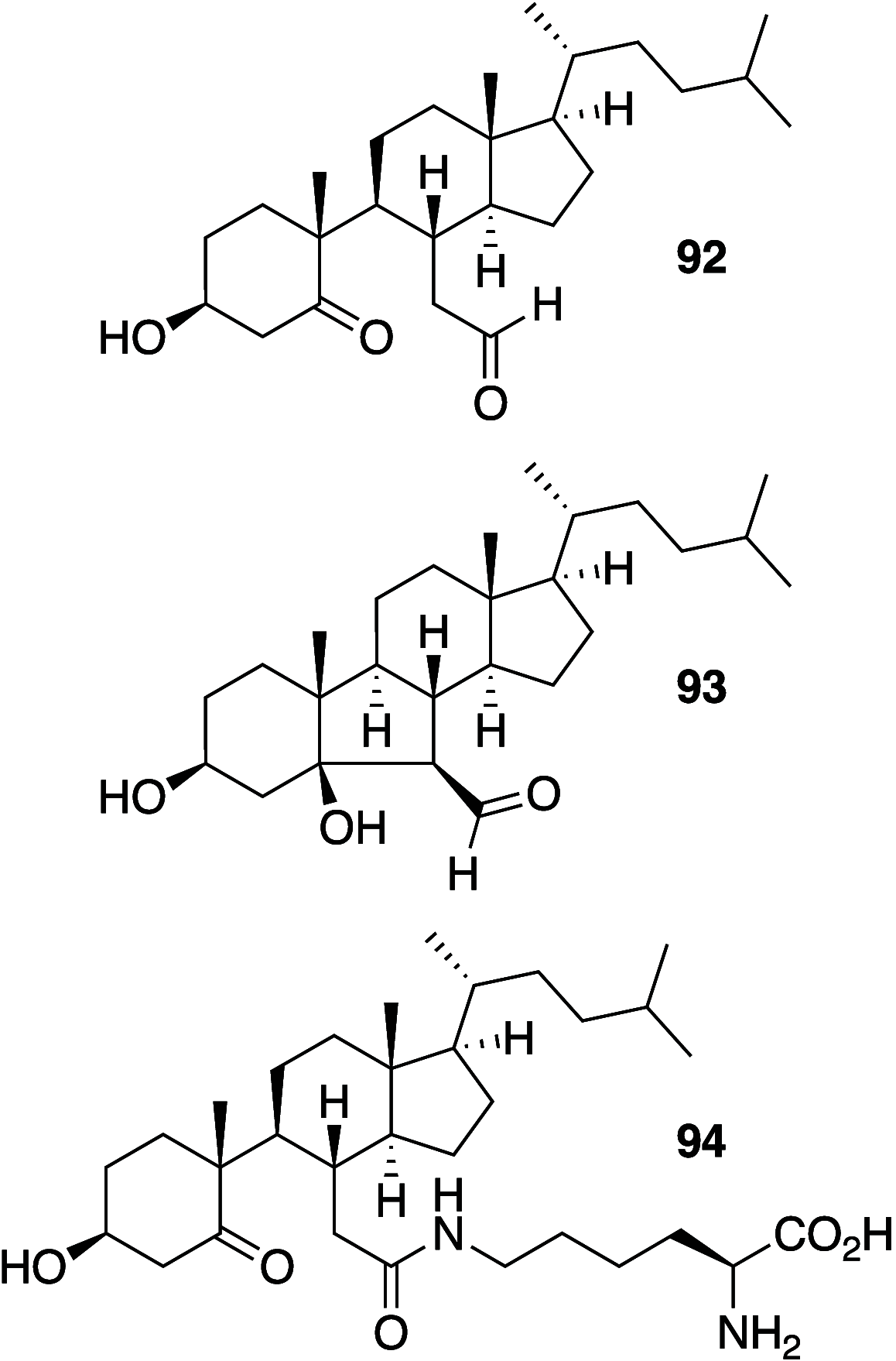
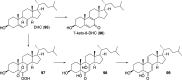
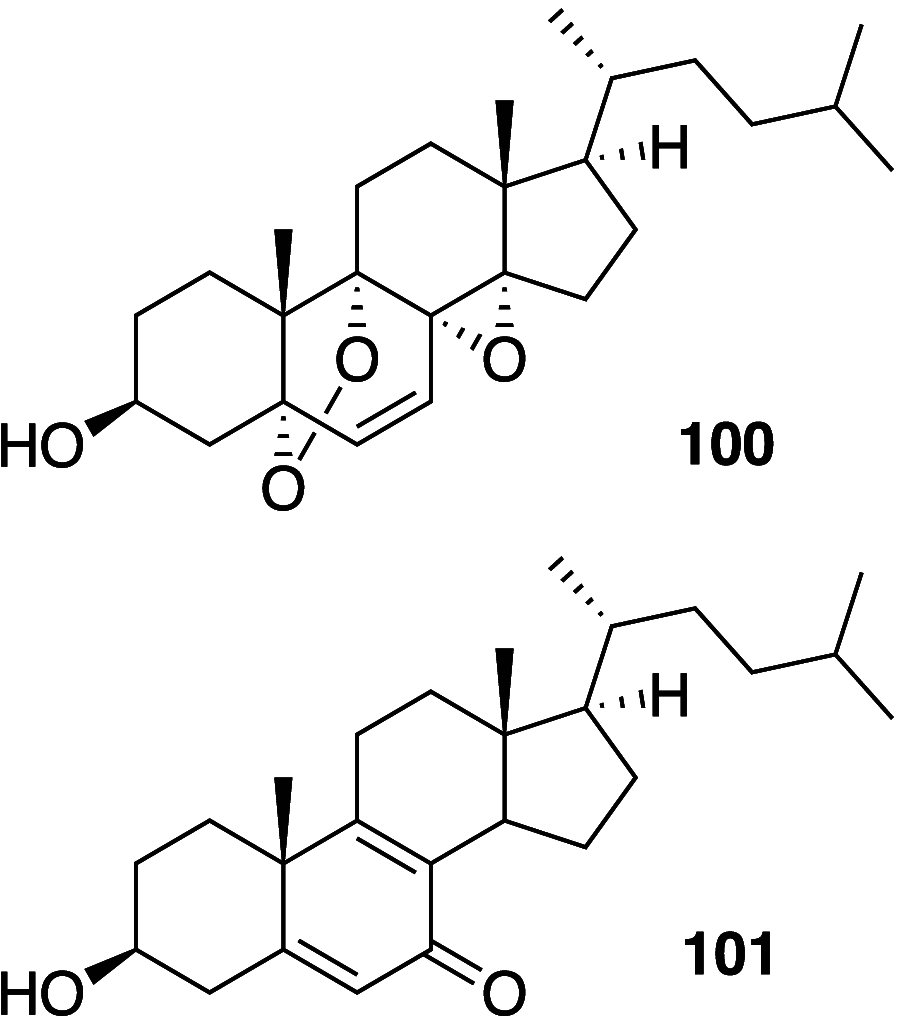
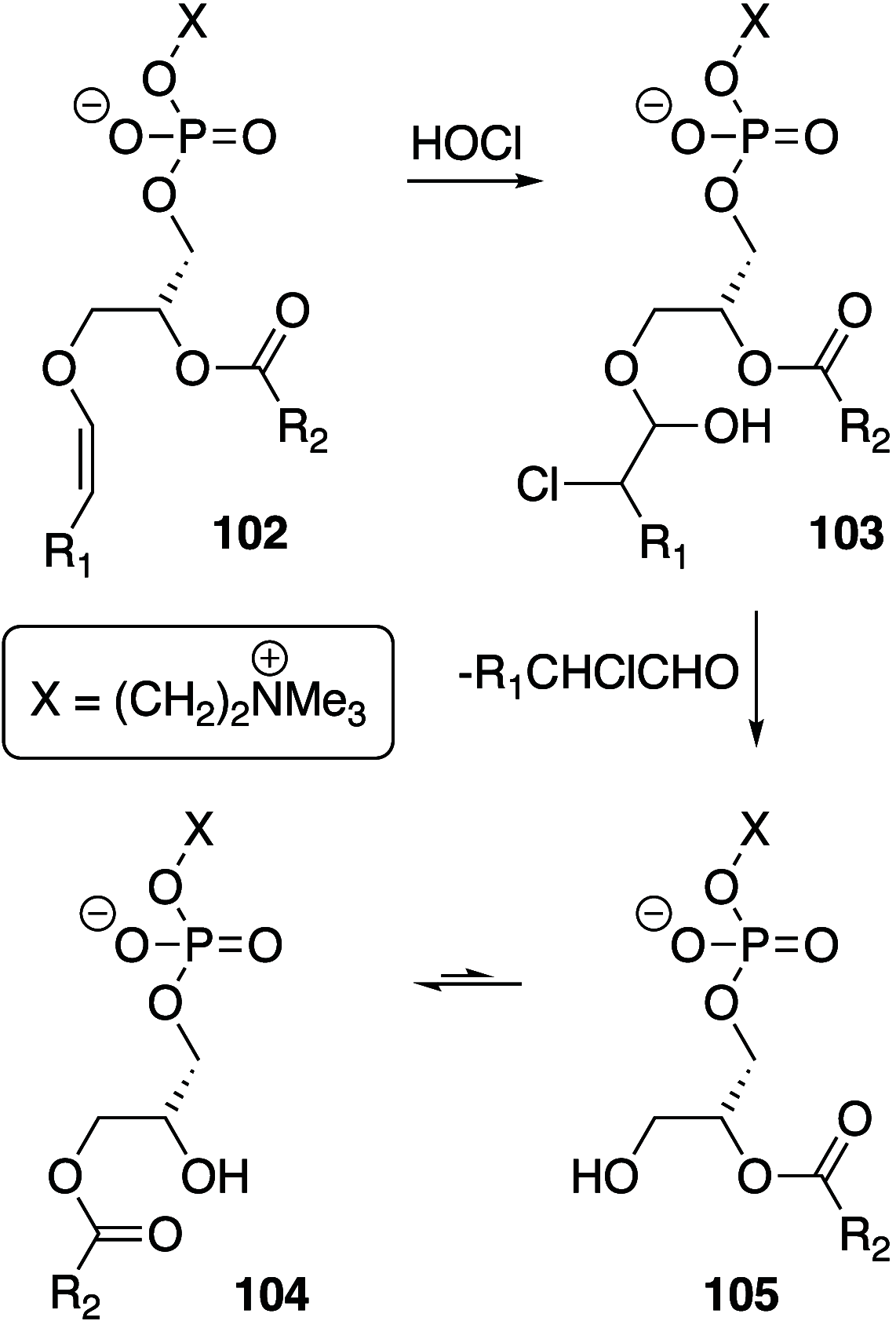
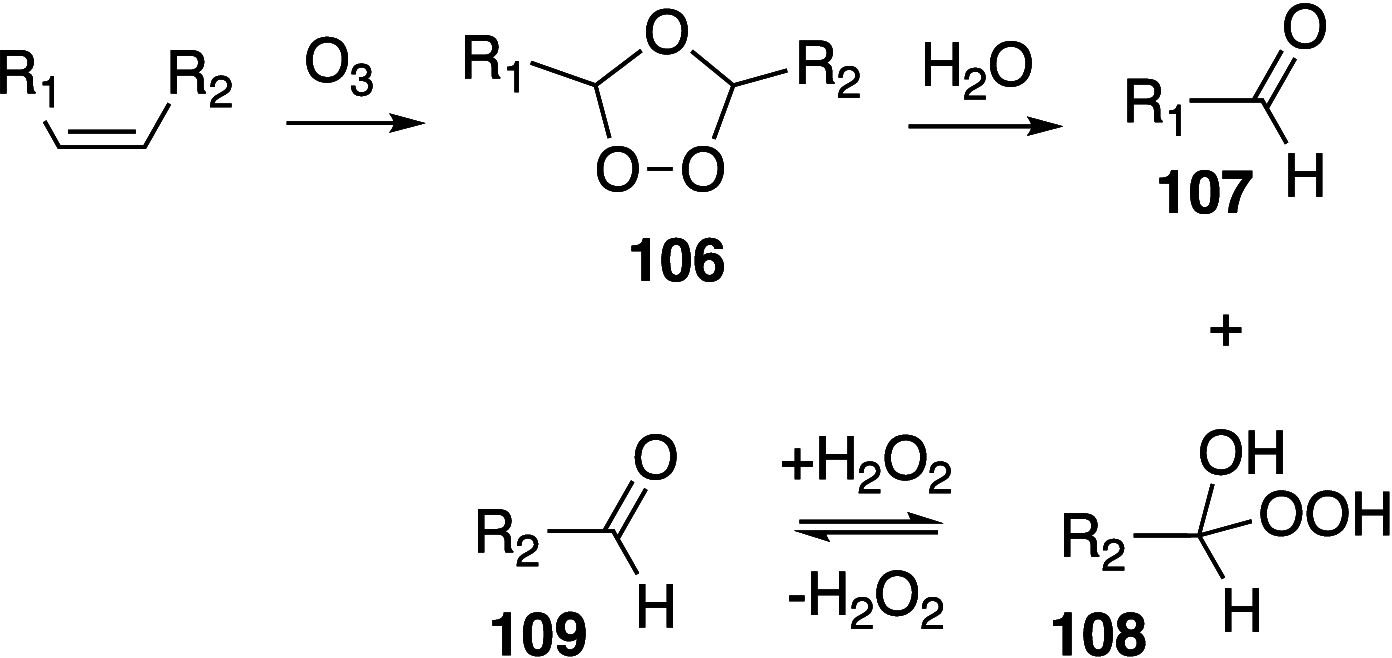
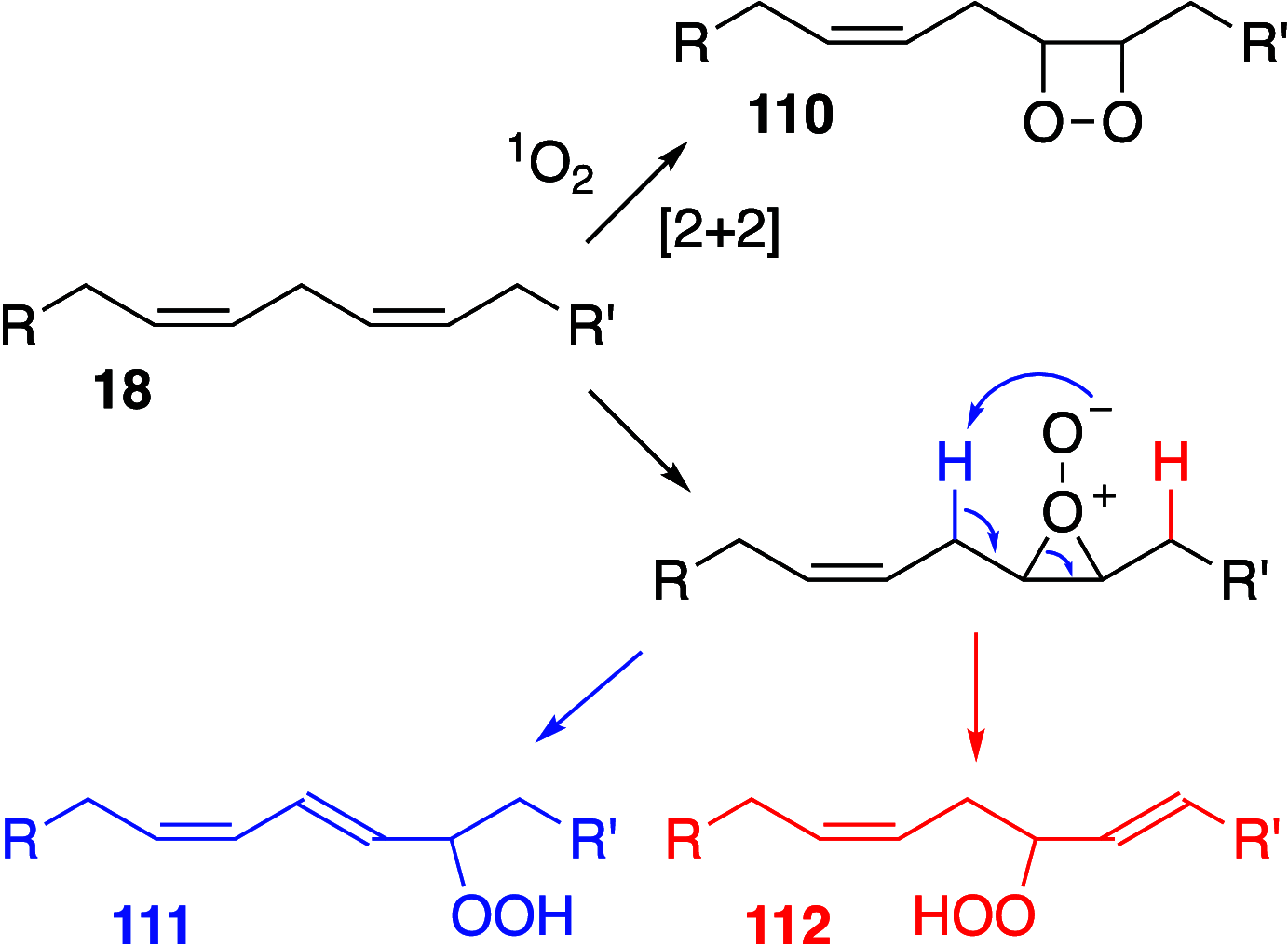
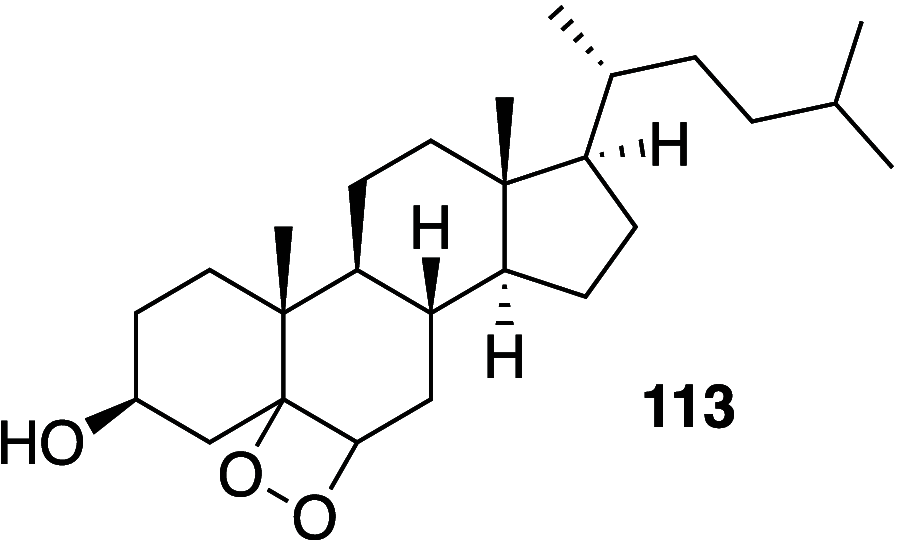
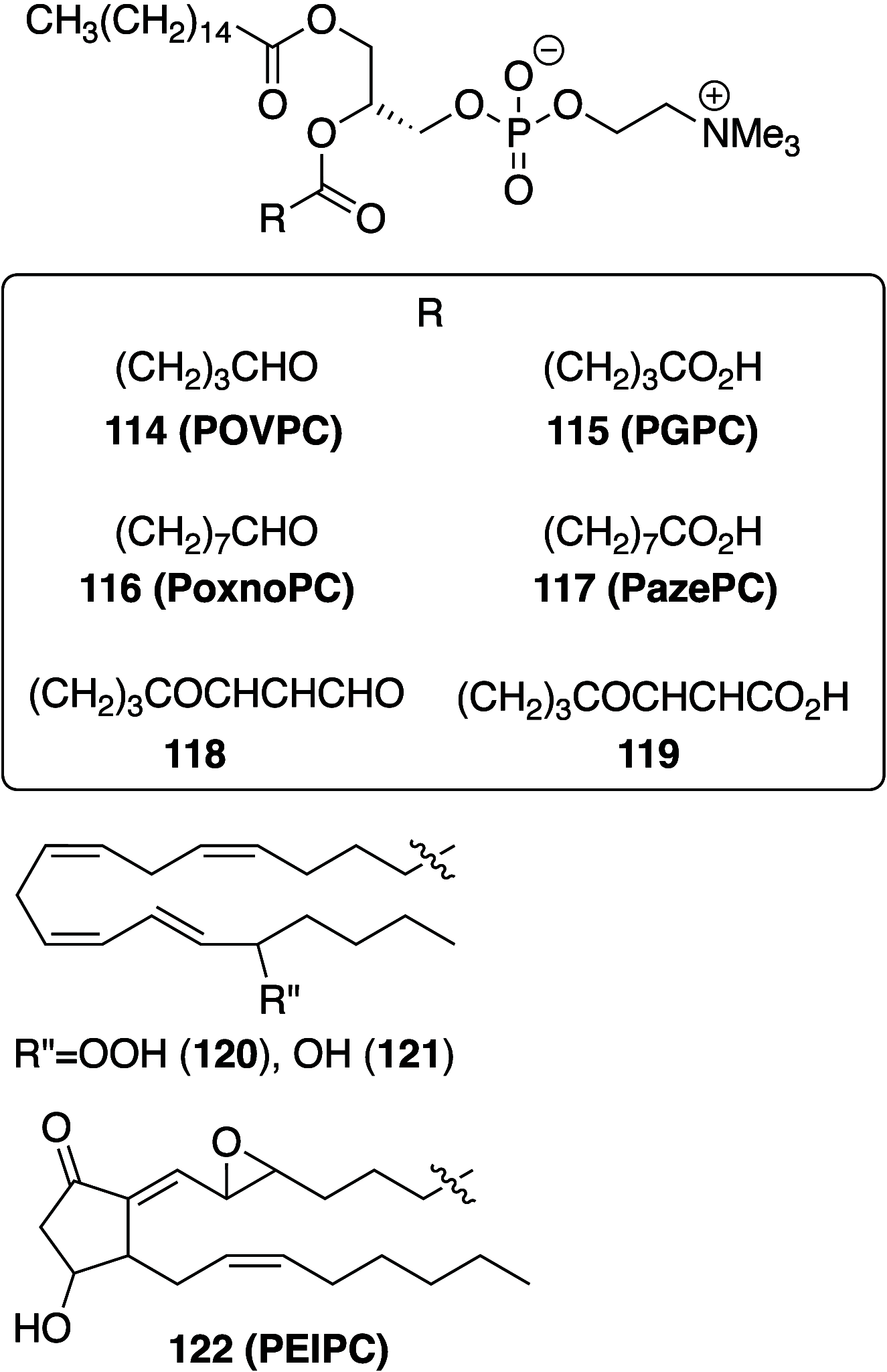
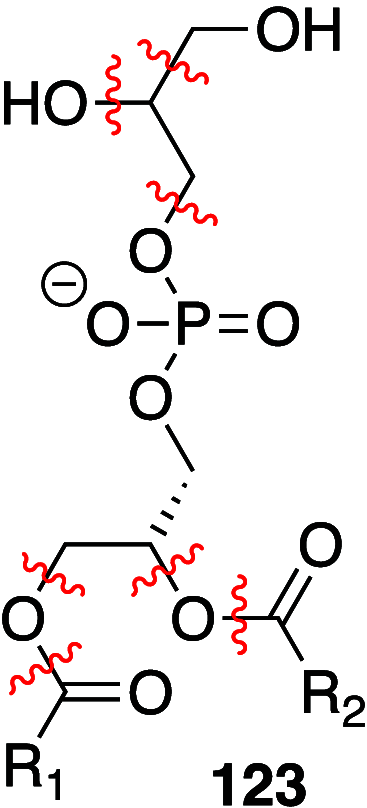
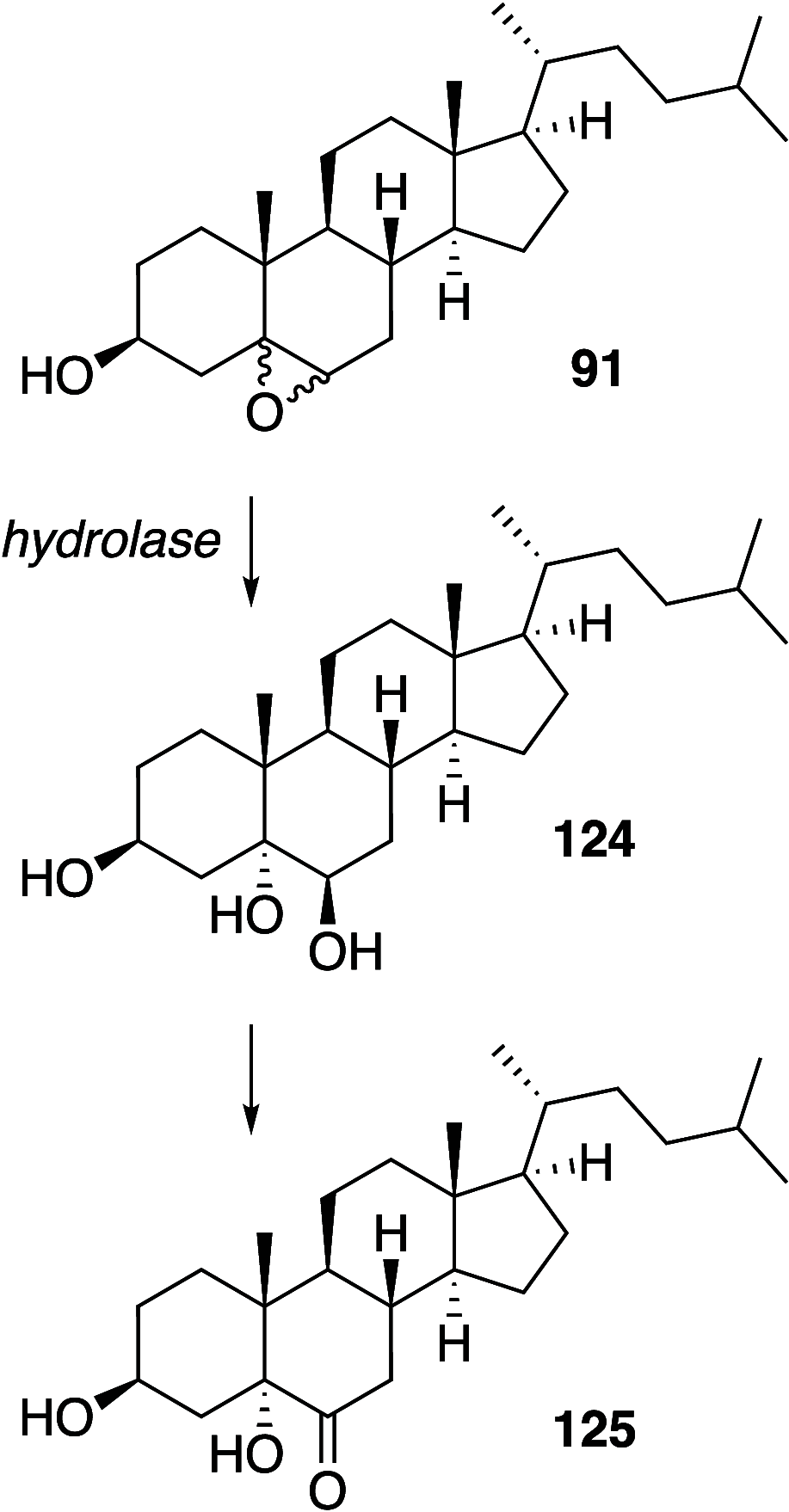
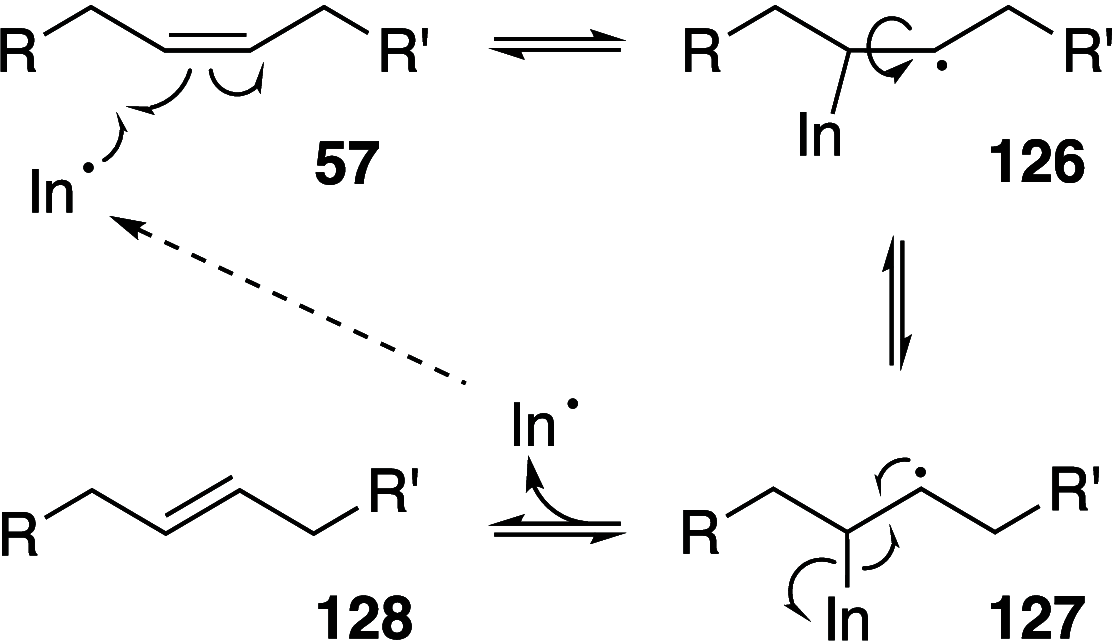
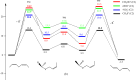
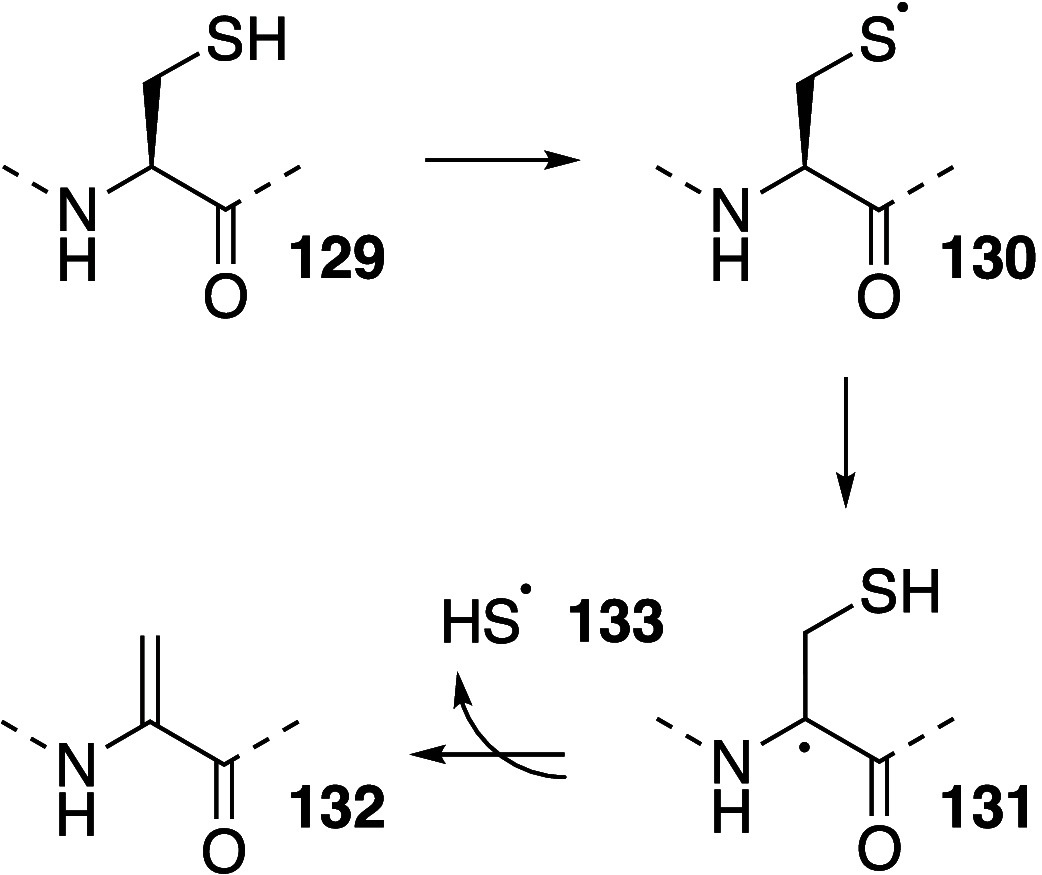
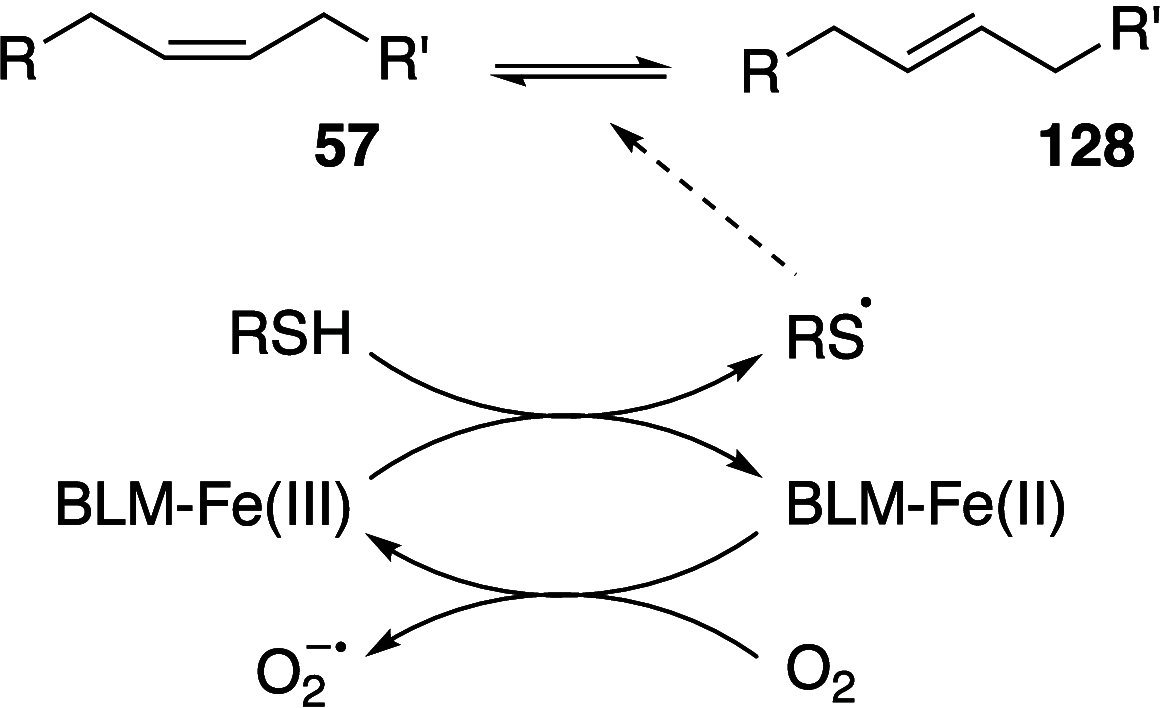
Similar articles
-
Planning Implications Related to Sterilization-Sensitive Science Investigations Associated with Mars Sample Return (MSR).Astrobiology. 2022 Jun;22(S1):S112-S164. doi: 10.1089/AST.2021.0113. Epub 2022 May 19. Astrobiology. 2022. PMID: 34904892
-
Elastic deformation of membrane bilayers probed by deuterium NMR relaxation.J Am Chem Soc. 2002 Jul 17;124(28):8471-84. doi: 10.1021/ja012660p. J Am Chem Soc. 2002. PMID: 12105929
-
The importance of membrane defects-lessons from simulations.Acc Chem Res. 2014 Aug 19;47(8):2244-51. doi: 10.1021/ar4002729. Epub 2014 Jun 3. Acc Chem Res. 2014. PMID: 24892900
-
Structure and functional properties of diacylglycerols in membranes.Prog Lipid Res. 1999 Jan;38(1):1-48. doi: 10.1016/s0163-7827(98)00021-6. Prog Lipid Res. 1999. PMID: 10396601 Review.
-
Structural and functional properties of hydration and confined water in membrane interfaces.Biochim Biophys Acta. 2008 Dec;1778(12):2655-70. doi: 10.1016/j.bbamem.2008.08.025. Epub 2008 Sep 12. Biochim Biophys Acta. 2008. PMID: 18834854 Review.
Cited by
-
Harnessing the power of bee venom for therapeutic and regenerative medical applications: an updated review.Front Pharmacol. 2024 Jul 18;15:1412245. doi: 10.3389/fphar.2024.1412245. eCollection 2024. Front Pharmacol. 2024. PMID: 39092234 Free PMC article. Review.
References
-
- Robertson J. D. The Ultrastructure of Cell Membranes and Their Derivatives. Biochem. Soc. Symp. 1959, 16, 3–43. - PubMed
-
- Danielli J. F.; Davson H. A Contribution to the Theory of Permeability of Thin Films. J. Cell. Comp. Physiol. 1935, 5, 495–508. 10.1002/jcp.1030050409. - DOI
-
- Gregoriadis G. Liposomes and mRNA: Two Technologies Together Create a Covid-19 Vaccine. Med. Drug Discovery 2021, 12, 100104.10.1016/j.medidd.2021.100104. - DOI
-
- Le M. T.; Litzenberger J. K.; Prenner E. J.. Biomimetic Model Membrane Systems Serve as Increasingly Valuable in Vitro Tools. In Advances in Biomimetics; George A., Ed.; IntechOpen: London, UK, 2011; pp 215–276.
Publication types
MeSH terms
Substances
LinkOut - more resources
Full Text Sources