Rapid evolution of an adaptive multicellular morphology of Candida auris during systemic infection
- PMID: 38493178
- PMCID: PMC10944540
- DOI: 10.1038/s41467-024-46786-8
Rapid evolution of an adaptive multicellular morphology of Candida auris during systemic infection
Abstract
Candida auris has become a serious threat to public health. The mechanisms of how this fungal pathogen adapts to the mammalian host are poorly understood. Here we report the rapid evolution of an adaptive C. auris multicellular aggregative morphology in the murine host during systemic infection. C. auris aggregative cells accumulate in the brain and exhibit obvious advantages over the single-celled yeast-form cells during systemic infection. Genetic mutations, specifically de novo point mutations in genes associated with cell division or budding processes, underlie the rapid evolution of this aggregative phenotype. Most mutated C. auris genes are associated with the regulation of cell wall integrity, cytokinesis, cytoskeletal properties, and cellular polarization. Moreover, the multicellular aggregates are notably more recalcitrant to the host antimicrobial peptides LL-37 and PACAP relative to the single-celled yeast-form cells. Overall, to survive in the host, C. auris can rapidly evolve a multicellular aggregative morphology via genetic mutations.
© 2024. The Author(s).
Conflict of interest statement
Clarissa J. Nobile is a cofounder of BioSynesis, Inc., a company developing diagnostics and therapeutics for biofilm infections. All other authors declare no conflicts of interest.
Figures
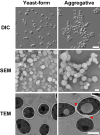
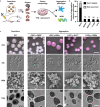
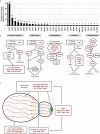
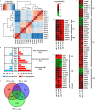
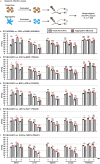
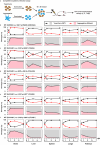
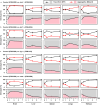
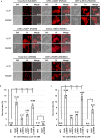
Similar articles
-
Cell aggregation mediated by ACE2 deletion in Candida auris modulates fungal colonization and host immune responses in the skin.mSphere. 2024 Nov 21;9(11):e0073424. doi: 10.1128/msphere.00734-24. Epub 2024 Oct 30. mSphere. 2024. PMID: 39475280 Free PMC article.
-
Candida auris Phenotypic Heterogeneity Determines Pathogenicity In Vitro.mSphere. 2020 Jun 24;5(3):e00371-20. doi: 10.1128/mSphere.00371-20. mSphere. 2020. PMID: 32581078 Free PMC article.
-
Drosophila melanogaster as a Rapid and Reliable In Vivo Infection Model to Study the Emerging Yeast Pathogen Candida auris.Methods Mol Biol. 2022;2517:299-316. doi: 10.1007/978-1-0716-2417-3_24. Methods Mol Biol. 2022. PMID: 35674964
-
Host defense mechanisms against Candida auris.Expert Rev Anti Infect Ther. 2023 Jul-Dec;21(10):1087-1096. doi: 10.1080/14787210.2023.2264500. Epub 2023 Oct 27. Expert Rev Anti Infect Ther. 2023. PMID: 37753840 Review.
-
Intra-clade Heterogeneity in Candida auris: Risk of Management.Curr Microbiol. 2023 Jul 24;80(9):295. doi: 10.1007/s00284-023-03416-8. Curr Microbiol. 2023. PMID: 37486431 Review.
Cited by
-
Insight into Virulence and Mechanisms of Amphotericin B Resistance in the Candida haemulonii Complex.J Fungi (Basel). 2024 Aug 28;10(9):615. doi: 10.3390/jof10090615. J Fungi (Basel). 2024. PMID: 39330375 Free PMC article. Review.
-
Going fishing: how to get what you want from a fungal genetic screen.mSphere. 2024 Jul 30;9(7):e0063823. doi: 10.1128/msphere.00638-23. Epub 2024 Jul 3. mSphere. 2024. PMID: 38958459 Free PMC article. Review.
-
Evolutionary accumulation of FKS1 mutations from clinical echinocandin-resistant Candida auris.Emerg Microbes Infect. 2024 Dec;13(1):2377584. doi: 10.1080/22221751.2024.2377584. Epub 2024 Jul 22. Emerg Microbes Infect. 2024. PMID: 38989545 Free PMC article.
-
Staphylococcus aureus SaeRS impairs macrophage immune functions through bacterial clumps formation in the early stage of infection.NPJ Biofilms Microbiomes. 2024 Oct 6;10(1):102. doi: 10.1038/s41522-024-00576-8. NPJ Biofilms Microbiomes. 2024. PMID: 39370453 Free PMC article.
-
Suppressing the virulence factors of Candida auris with baicalein through multifaceted mechanisms.Arch Microbiol. 2024 Jul 11;206(8):349. doi: 10.1007/s00203-024-04038-9. Arch Microbiol. 2024. PMID: 38992278
References
-
- WHO. WHO Fungal Priority Pathogens List to Guide Research, Development and Public Health Action. WHO https://www.who.int/publications/i/item/9789240060241 (2022).
MeSH terms
Substances
Grants and funding
- 31930005 and 82272359/National Natural Science Foundation of China (National Science Foundation of China)
- 32170193 and 32000018/National Natural Science Foundation of China (National Science Foundation of China)
- 32170193 and 32000018/National Natural Science Foundation of China (National Science Foundation of China)
- R35GM124594/U.S. Department of Health & Human Services | NIH | National Institute of General Medical Sciences (NIGMS)