Enhancer selectivity in space and time: from enhancer-promoter interactions to promoter activation
- PMID: 38413840
- PMCID: PMC11574175
- DOI: 10.1038/s41580-024-00710-6
Enhancer selectivity in space and time: from enhancer-promoter interactions to promoter activation
Abstract
The primary regulators of metazoan gene expression are enhancers, originally functionally defined as DNA sequences that can activate transcription at promoters in an orientation-independent and distance-independent manner. Despite being crucial for gene regulation in animals, what mechanisms underlie enhancer selectivity for promoters, and more fundamentally, how enhancers interact with promoters and activate transcription, remain poorly understood. In this Review, we first discuss current models of enhancer-promoter interactions in space and time and how enhancers affect transcription activation. Next, we discuss different mechanisms that mediate enhancer selectivity, including repression, biochemical compatibility and regulation of 3D genome structure. Through 3D polymer simulations, we illustrate how the ability of 3D genome folding mechanisms to mediate enhancer selectivity strongly varies for different enhancer-promoter interaction mechanisms. Finally, we discuss how recent technical advances may provide new insights into mechanisms of enhancer-promoter interactions and how technical biases in methods such as Hi-C and Micro-C and imaging techniques may affect their interpretation.
© 2024. Springer Nature Limited.
Conflict of interest statement
Competing interests
The authors declare no competing interests.
Figures
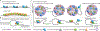
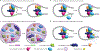
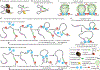
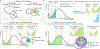
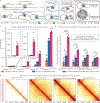
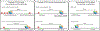
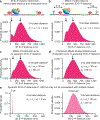
Similar articles
-
Three-dimensional genome architectural CCCTC-binding factor makes choice in duplicated enhancers at Pcdhα locus.Sci China Life Sci. 2020 Jun;63(6):835-844. doi: 10.1007/s11427-019-1598-4. Epub 2020 Apr 2. Sci China Life Sci. 2020. PMID: 32249388
-
Increased enhancer-promoter interactions during developmental enhancer activation in mammals.Nat Genet. 2024 Apr;56(4):675-685. doi: 10.1038/s41588-024-01681-2. Epub 2024 Mar 20. Nat Genet. 2024. PMID: 38509385 Free PMC article.
-
Characterization of genome-wide enhancer-promoter interactions reveals co-expression of interacting genes and modes of higher order chromatin organization.Cell Res. 2012 Mar;22(3):490-503. doi: 10.1038/cr.2012.15. Epub 2012 Jan 24. Cell Res. 2012. PMID: 22270183 Free PMC article.
-
The role of loop extrusion in enhancer-mediated gene activation.Curr Opin Genet Dev. 2023 Apr;79:102022. doi: 10.1016/j.gde.2023.102022. Epub 2023 Feb 24. Curr Opin Genet Dev. 2023. PMID: 36842325 Review.
-
Nuclear compartments, genome folding, and enhancer-promoter communication.Int Rev Cell Mol Biol. 2015;315:183-244. doi: 10.1016/bs.ircmb.2014.11.004. Epub 2015 Feb 9. Int Rev Cell Mol Biol. 2015. PMID: 25708464 Review.
Cited by
-
Dynamics of microcompartment formation at the mitosis-to-G1 transition.bioRxiv [Preprint]. 2024 Sep 16:2024.09.16.611917. doi: 10.1101/2024.09.16.611917. bioRxiv. 2024. PMID: 39345388 Free PMC article. Preprint.
-
Reversal of high-glucose-induced transcriptional and epigenetic memories through NRF2 pathway activation.Life Sci Alliance. 2024 May 16;7(8):e202302382. doi: 10.26508/lsa.202302382. Print 2024 Aug. Life Sci Alliance. 2024. PMID: 38755006 Free PMC article.
-
Transcription decouples estrogen-dependent changes in enhancer-promoter contact frequencies and spatial proximity.PLoS Genet. 2024 May 23;20(5):e1011277. doi: 10.1371/journal.pgen.1011277. eCollection 2024 May. PLoS Genet. 2024. PMID: 38781242 Free PMC article.
-
Molecular models of bidirectional promoter regulation.Curr Opin Struct Biol. 2024 Aug;87:102865. doi: 10.1016/j.sbi.2024.102865. Epub 2024 Jun 20. Curr Opin Struct Biol. 2024. PMID: 38905929 Free PMC article. Review.
-
A PRE loop at the dac locus acts as a topological chromatin structure that restricts and specifies enhancer-promoter communication.Nat Struct Mol Biol. 2024 Dec;31(12):1942-1954. doi: 10.1038/s41594-024-01375-7. Epub 2024 Aug 16. Nat Struct Mol Biol. 2024. PMID: 39152239 Free PMC article.
References
-
- Field A & Adelman K Evaluating enhancer function and transcription. Annu. Rev. Biochem. 89, 213–234 (2020). - PubMed
Publication types
MeSH terms
Substances
Grants and funding
LinkOut - more resources
Full Text Sources