HBV integrations reshaping genomic structures promote hepatocellular carcinoma
- PMID: 38395437
- PMCID: PMC11187386
- DOI: 10.1136/gutjnl-2023-330414
HBV integrations reshaping genomic structures promote hepatocellular carcinoma
Abstract
Objective: Hepatitis B virus (HBV)-related hepatocellular carcinoma (HCC), mostly characterised by HBV integrations, is prevalent worldwide. Previous HBV studies mainly focused on a few hotspot integrations. However, the oncogenic role of the other HBV integrations remains unclear. This study aimed to elucidate HBV integration-induced tumourigenesis further.
Design: Here, we illuminated the genomic structures encompassing HBV integrations in 124 HCCs across ages using whole genome sequencing and Nanopore long reads. We classified a repertoire of integration patterns featured by complex genomic rearrangement. We also conducted a clustered regularly interspaced short palindromic repeat (CRISPR)-based gain-of-function genetic screen in mouse hepatocytes. We individually activated each candidate gene in the mouse model to uncover HBV integration-mediated oncogenic aberration that elicits tumourigenesis in mice.
Results: These HBV-mediated rearrangements are significantly enriched in a bridge-fusion-bridge pattern and interchromosomal translocations, and frequently led to a wide range of aberrations including driver copy number variations in chr 4q, 5p (TERT), 6q, 8p, 16q, 9p (CDKN2A/B), 17p (TP53) and 13q (RB1), and particularly, ultra-early amplifications in chr8q. Integrated HBV frequently contains complex structures correlated with the translocation distance. Paired breakpoints within each integration event usually exhibit different microhomology, likely mediated by different DNA repair mechanisms. HBV-mediated rearrangements significantly correlated with young age, higher HBV DNA level and TP53 mutations but were less prevalent in the patients subjected to prior antiviral therapies. Finally, we recapitulated the TONSL and TMEM65 amplification in chr8q led by HBV integration using CRISPR/Cas9 editing and demonstrated their tumourigenic potentials.
Conclusion: HBV integrations extensively reshape genomic structures and promote hepatocarcinogenesis (graphical abstract), which may occur early in a patient's life.
Keywords: CARCINOGENESIS; GENE MUTATION; HEPATOCELLULAR CARCINOMA.
© Author(s) (or their employer(s)) 2024. Re-use permitted under CC BY-NC. No commercial re-use. See rights and permissions. Published by BMJ.
Conflict of interest statement
Competing interests: None declared.
Figures
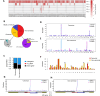
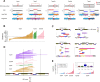
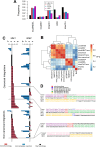
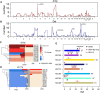
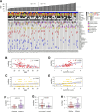
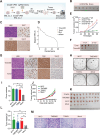
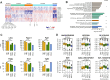
Similar articles
-
Hepatitis B virus integrations promote local and distant oncogenic driver alterations in hepatocellular carcinoma.Gut. 2022 Mar;71(3):616-626. doi: 10.1136/gutjnl-2020-323153. Epub 2021 Feb 9. Gut. 2022. PMID: 33563643 Free PMC article.
-
Molecular cytogenetic evaluation of virus-associated and non-viral hepatocellular carcinoma: analysis of 26 carcinomas and 12 concurrent dysplasias.J Pathol. 2000 Oct;192(2):207-15. doi: 10.1002/1096-9896(2000)9999:9999<::AID-PATH690>3.0.CO;2-#. J Pathol. 2000. PMID: 11004697
-
Targeted Long-Read Sequencing Reveals Comprehensive Architecture, Burden, and Transcriptional Signatures from Hepatitis B Virus-Associated Integrations and Translocations in Hepatocellular Carcinoma Cell Lines.J Virol. 2021 Sep 9;95(19):e0029921. doi: 10.1128/JVI.00299-21. Epub 2021 Jul 21. J Virol. 2021. PMID: 34287049 Free PMC article.
-
Role of hepatitis B virus DNA integration in human hepatocarcinogenesis.World J Gastroenterol. 2014 May 28;20(20):6236-43. doi: 10.3748/wjg.v20.i20.6236. World J Gastroenterol. 2014. PMID: 24876744 Free PMC article. Review.
-
Hepatitis B Virus DNA Integration Drives Carcinogenesis and Provides a New Biomarker for HBV-related HCC.Cell Mol Gastroenterol Hepatol. 2023;15(4):921-929. doi: 10.1016/j.jcmgh.2023.01.001. Epub 2023 Jan 20. Cell Mol Gastroenterol Hepatol. 2023. PMID: 36690297 Free PMC article. Review.
Cited by
-
Applications of CRISPR/Cas as a Toolbox for Hepatitis B Virus Detection and Therapeutics.Viruses. 2024 Oct 2;16(10):1565. doi: 10.3390/v16101565. Viruses. 2024. PMID: 39459899 Free PMC article. Review.
-
Applications of cell therapy in the treatment of virus-associated cancers.Nat Rev Clin Oncol. 2024 Oct;21(10):709-724. doi: 10.1038/s41571-024-00930-x. Epub 2024 Aug 19. Nat Rev Clin Oncol. 2024. PMID: 39160243 Review.
-
Extended analysis on peripheral blood cytokines correlated with hepatitis B virus viral load in chronically infected patients - a systematic review and meta-analysis.Front Med (Lausanne). 2024 Jul 31;11:1429926. doi: 10.3389/fmed.2024.1429926. eCollection 2024. Front Med (Lausanne). 2024. PMID: 39149606 Free PMC article.
-
The role of the hepatitis B virus genome and its integration in the hepatocellular carcinoma.Front Microbiol. 2024 Sep 6;15:1469016. doi: 10.3389/fmicb.2024.1469016. eCollection 2024. Front Microbiol. 2024. PMID: 39309526 Free PMC article. Review.
-
Leveraging oncovirus-derived antigen against the viral malignancies in adoptive cell therapies.Biomark Res. 2024 Jul 29;12(1):71. doi: 10.1186/s40364-024-00617-6. Biomark Res. 2024. PMID: 39075601 Free PMC article. Review.
References
Publication types
MeSH terms
LinkOut - more resources
Full Text Sources
Medical
Research Materials
Miscellaneous