Preparation of bilayer tissue-engineered polyurethane/poly-L-lactic acid nerve conduits and their in vitro characterization for use in peripheral nerve regeneration
- PMID: 38388447
- PMCID: PMC10885435
- DOI: 10.1186/s13036-024-00412-9
Preparation of bilayer tissue-engineered polyurethane/poly-L-lactic acid nerve conduits and their in vitro characterization for use in peripheral nerve regeneration
Abstract
Background: Due to loss of peripheral nerve structure and/or function resulting from trauma, accidents, and other causes, peripheral nerve injuries continue to be a major clinical problem. These injuries can cause partial or total loss of sensory, motor, and autonomic capabilities as well as neuropathic pain. PNI affects between 13 and 23 out of every 100,000 people annually in developed countries. Regeneration of damaged nerves and restoration of function after peripheral nerve injury remain significant therapeutic challenges. Although autologous nerve graft transplantation is a viable therapy option in several clinical conditions, donor site morbidity and a lack of donor tissue often hinder full functional recovery. Biomimetic conduits used in tissue engineering to encourage and direct peripheral nerve regeneration by providing a suitable microenvironment for nerve ingrowth are only one example of the cutting-edge methods made possible by this field. Many innate extracellular matrix (ECM) structures of different tissues can be successfully mimicked by nanofibrous scaffolds. Nanofibrous scaffolds can closely mimic the surface structure and morphology of native ECMs of many tissues.
Methods: In this study, we have produced bilayer nanofibrous nerve conduit based on poly-lactic acid/polyurethane/multiwall carbon nanotube (PLA/PU/MWCNT), for application as composite scaffolds for static nerve tissue engineering. The contact angle was indicated to show the hydrophilicity properties of electrospun nanofibers. The SEM images were analyzed to determine the fiber's diameters, scaffold morphology, and endometrial stem cell adhesion. Moreover, MTT assay and DAPI staining were used to show the viability and proliferation of endometrial stem cells.
Results: The constructed bilayer PLA/PU/MWCNT scaffolds demonstrated the capacity to support cell attachment, and the vitality of samples was assessed using SEM, MTT assay, and DAPI staining technique.
Conclusions: According to an in vitro study, electrospun bilayer PLA/PU/MWCNT scaffolds can encourage the adhesion and proliferation of human endometrial stem cells (hEnSCs) and create the ideal environment for increasing cell survival.
Keywords: Carbon nanotube; Human endometrial stem cells; Nanofibrous scaffolds; Nerve conduit; Neural differentiation; Poly-L-lactic acid; Polyurethane; Sciatic nerve injury; Tissue engineering.
© 2024. The Author(s).
Conflict of interest statement
The authors declare no competing interests.
Figures
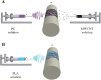
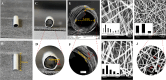
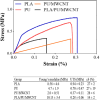
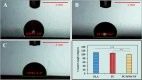
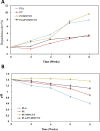
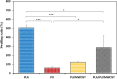
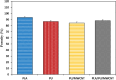
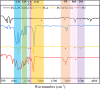
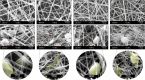
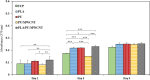
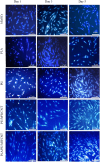
Similar articles
-
Preparation of fibrin gel scaffolds containing MWCNT/PU nanofibers for neural tissue engineering.J Biomed Mater Res A. 2019 Apr;107(4):802-814. doi: 10.1002/jbm.a.36596. Epub 2019 Jan 11. J Biomed Mater Res A. 2019. PMID: 30578713
-
Electrospun nerve guide scaffold of poly(ε-caprolactone)/collagen/nanobioglass: an in vitro study in peripheral nerve tissue engineering.J Biomed Mater Res A. 2017 Jul;105(7):1960-1972. doi: 10.1002/jbm.a.36068. Epub 2017 Apr 3. J Biomed Mater Res A. 2017. PMID: 28324629
-
Novel electrospun conduit based on polyurethane/collagen enhanced by nanobioglass for peripheral nerve tissue engineering.J Biomater Sci Polym Ed. 2022 Apr;33(6):801-822. doi: 10.1080/09205063.2021.2021350. Epub 2022 Jan 7. J Biomater Sci Polym Ed. 2022. PMID: 34983332
-
Harnessing Nanotopography of Electrospun Nanofibrous Nerve Guide Conduits (NGCs) for Neural Tissue Engineering.Adv Exp Med Biol. 2018;1078:395-408. doi: 10.1007/978-981-13-0950-2_20. Adv Exp Med Biol. 2018. PMID: 30357634 Review.
-
Biomechanical microenvironment in peripheral nerve regeneration: from pathophysiological understanding to tissue engineering development.Theranostics. 2022 Jun 27;12(11):4993-5014. doi: 10.7150/thno.74571. eCollection 2022. Theranostics. 2022. PMID: 35836812 Free PMC article. Review.
Cited by
-
Natural Compounds and Biomimetic Engineering to Influence Fibroblast Behavior in Wound Healing.Int J Mol Sci. 2024 Mar 14;25(6):3274. doi: 10.3390/ijms25063274. Int J Mol Sci. 2024. PMID: 38542247 Free PMC article. Review.
References
-
- Reyes O, Kuffler DP. Promoting neurological recovery following a traumatic peripheral nerve injury. P R Health Sci J. 2005;24(3):215–224. - PubMed
LinkOut - more resources
Full Text Sources