Lutetium texaphyrin: A photocatalyst that triggers pyroptosis via biomolecular photoredox catalysis
- PMID: 38381784
- PMCID: PMC10907263
- DOI: 10.1073/pnas.2314620121
Lutetium texaphyrin: A photocatalyst that triggers pyroptosis via biomolecular photoredox catalysis
Abstract
Photon-controlled pyroptosis activation (PhotoPyro) is a promising technique for cancer immunotherapy due to its noninvasive nature, precise control, and ease of operation. Here, we report that biomolecular photoredox catalysis in cells might be an important mechanism underlying PhotoPyro. Our findings reveal that the photocatalyst lutetium texaphyrin (MLu) facilitates rapid and direct photoredox oxidation of nicotinamide adenine dinucleotide, nicotinamide adenine dinucleotide phosphate, and various amino acids, thereby triggering pyroptosis through the caspase 3/GSDME pathway. This mechanism is distinct from the well-established role of MLu as a photodynamic therapy sensitizer in cells. Two analogs of MLu, bearing different coordinated central metal cations, were also explored as controls. The first control, gadolinium texaphyrin (MGd), is a weak photocatalyst but generates reactive oxygen species (ROS) efficiently. The second control, manganese texaphyrin (MMn), is ineffective as both a photocatalyst and a ROS generator. Neither MGd nor MMn was found to trigger pyroptosis under the conditions where MLu was active. Even in the presence of a ROS scavenger, treating MDA-MB-231 cells with MLu at concentrations as low as 50 nM still allows for pyroptosis photo-activation. The present findings highlight how biomolecular photoredox catalysis could contribute to pyroptosis activation by mechanisms largely independent of ROS.
Keywords: NAD(P)H; NIR photocatalyst; PhotoPyro; photoredox catalysis; pyroptosis.
Conflict of interest statement
Competing interests statement:J.L.S. is a cofounder of a new startup, InnovoTEX, Inc. As yet, the position in uncompensated, either with money or stock. However, in the near future an equity grant is anticipated. No funding from InnovoTEX comes to J.L.S.’s or any of the authors’ laboratories.
Figures
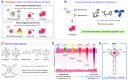
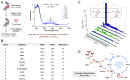
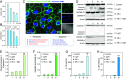
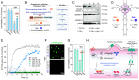
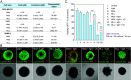
Similar articles
-
4,5-Diethyl-10,23-dimethyl-9,24-bis(3-hydroxypropyl)-16,-17-bis(3,hydroxypropyl)oxy-13,20,25,26,27—pentaazapentacyclo-[20.2.1.13,6.19,11.014,19]heptacosa-3,5,8,10,12,14,16,18,20,22,24-undecaene gadolinium hydrates.2007 Dec 6 [updated 2008 Jan 8]. In: Molecular Imaging and Contrast Agent Database (MICAD) [Internet]. Bethesda (MD): National Center for Biotechnology Information (US); 2004–2013. 2007 Dec 6 [updated 2008 Jan 8]. In: Molecular Imaging and Contrast Agent Database (MICAD) [Internet]. Bethesda (MD): National Center for Biotechnology Information (US); 2004–2013. PMID: 20641453 Free Books & Documents. Review.
-
Photoredox catalysis may be a general mechanism in photodynamic therapy.Proc Natl Acad Sci U S A. 2022 Aug 23;119(34):e2210504119. doi: 10.1073/pnas.2210504119. Epub 2022 Aug 15. Proc Natl Acad Sci U S A. 2022. PMID: 35969782 Free PMC article.
-
Tom20 senses iron-activated ROS signaling to promote melanoma cell pyroptosis.Cell Res. 2018 Dec;28(12):1171-1185. doi: 10.1038/s41422-018-0090-y. Epub 2018 Oct 4. Cell Res. 2018. PMID: 30287942 Free PMC article.
-
Decitabine combined with cold atmospheric plasma induces pyroptosis via the ROS/Caspase-3/GSDME signaling pathway in Ovcar5 cells.Biochim Biophys Acta Gen Subj. 2024 Jun;1868(6):130602. doi: 10.1016/j.bbagen.2024.130602. Epub 2024 Mar 20. Biochim Biophys Acta Gen Subj. 2024. PMID: 38513927
-
Photon-Controlled Pyroptosis Activation (PhotoPyro): An Emerging Trigger for Antitumor Immune Response.J Am Chem Soc. 2023 Mar 22;145(11):6007-6023. doi: 10.1021/jacs.3c01231. Epub 2023 Mar 7. J Am Chem Soc. 2023. PMID: 36881923 Review.
Cited by
-
Trapped in Cells: A Selective Accumulation Approach for Type-I Photodynamic Ablation of Cancer Stem-like Cells.JACS Au. 2024 Aug 26;4(9):3657-3667. doi: 10.1021/jacsau.4c00642. eCollection 2024 Sep 23. JACS Au. 2024. PMID: 39328753 Free PMC article.
References
-
- Ryu K. A., Kaszuba C. M., Bissonnette N. B., Oslund R. C., Fadeyi O. O., Interrogating biological systems using visible-light-powered catalysis. Nat. Rev. Chem. 5, 322–337 (2021). - PubMed
-
- Xiong H., et al. , Photo-controllable biochemistry: Exploiting the photocages in phototherapeutic window. Chem 9, 29–64 (2023).
-
- Hüll K., Morstein J., Trauner D., In vivo photopharmacology. Chem. Rev. 118, 10710–10747 (2018). - PubMed
-
- Xu Y., et al. , Photodynamic Alzheimer’s disease therapy: From molecular catalysis to photo-nanomedicine. Coordin. Chem. Rev. 470, 214726 (2022).
-
- Li M., et al. , Superoxide radical photogenerator with amplification effect: Surmounting the Achilles’ heels of photodynamic oncotherapy. J. Am. Chem. Soc. 141, 2695–2702 (2019). - PubMed
MeSH terms
Substances
Grants and funding
LinkOut - more resources
Full Text Sources
Research Materials
Miscellaneous