Diversity, origin, and evolution of the ESCRT systems
- PMID: 38380930
- PMCID: PMC10936438
- DOI: 10.1128/mbio.00335-24
Diversity, origin, and evolution of the ESCRT systems
Abstract
Endosomal sorting complexes required for transport (ESCRT) play key roles in protein sorting between membrane-bounded compartments of eukaryotic cells. Homologs of many ESCRT components are identifiable in various groups of archaea, especially in Asgardarchaeota, the archaeal phylum that is currently considered to include the closest relatives of eukaryotes, but not in bacteria. We performed a comprehensive search for ESCRT protein homologs in archaea and reconstructed ESCRT evolution using the phylogenetic tree of Vps4 ATPase (ESCRT IV) as a scaffold and using sensitive protein sequence analysis and comparison of structural models to identify previously unknown ESCRT proteins. Several distinct groups of ESCRT systems in archaea outside of Asgard were identified, including proteins structurally similar to ESCRT-I and ESCRT-II, and several other domains involved in protein sorting in eukaryotes, suggesting an early origin of these components. Additionally, distant homologs of CdvA proteins were identified in Thermoproteales which are likely components of the uncharacterized cell division system in these archaea. We propose an evolutionary scenario for the origin of eukaryotic and Asgard ESCRT complexes from ancestral building blocks, namely, the Vps4 ATPase, ESCRT-III components, wH (winged helix-turn-helix fold) and possibly also coiled-coil, and Vps28-like domains. The last archaeal common ancestor likely encompassed a complex ESCRT system that was involved in protein sorting. Subsequent evolution involved either simplification, as in the TACK superphylum, where ESCRT was co-opted for cell division, or complexification as in Asgardarchaeota. In Asgardarchaeota, the connection between ESCRT and the ubiquitin system that was previously considered a eukaryotic signature was already established.IMPORTANCEAll eukaryotic cells possess complex intracellular membrane organization. Endosomal sorting complexes required for transport (ESCRT) play a central role in membrane remodeling which is essential for cellular functionality in eukaryotes. Recently, it has been shown that Asgard archaea, the archaeal phylum that includes the closest known relatives of eukaryotes, encode homologs of many components of the ESCRT systems. We employed protein sequence and structure comparisons to reconstruct the evolution of ESCRT systems in archaea and identified several previously unknown homologs of ESCRT subunits, some of which can be predicted to participate in cell division. The results of this reconstruction indicate that the last archaeal common ancestor already encoded a complex ESCRT system that was involved in protein sorting. In Asgard archaea, ESCRT systems evolved toward greater complexity, and in particular, the connection between ESCRT and the ubiquitin system that was previously considered a eukaryotic signature was established.
Keywords: Asgard archaea; ESCRT; cell division; membrane remodeling.
Conflict of interest statement
The authors declare no conflict of interest.
Figures
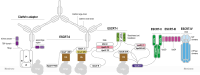
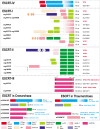
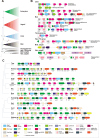
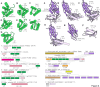
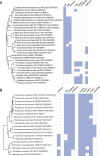
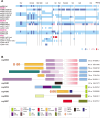
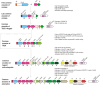
Update of
-
Diversity, Origin and Evolution of the ESCRT Systems.bioRxiv [Preprint]. 2024 Feb 7:2024.02.06.579148. doi: 10.1101/2024.02.06.579148. bioRxiv. 2024. Update in: mBio. 2024 Mar 13;15(3):e0033524. doi: 10.1128/mbio.00335-24. PMID: 38903064 Free PMC article. Updated. Preprint.
Similar articles
-
Diversity, Origin and Evolution of the ESCRT Systems.bioRxiv [Preprint]. 2024 Feb 7:2024.02.06.579148. doi: 10.1101/2024.02.06.579148. bioRxiv. 2024. Update in: mBio. 2024 Mar 13;15(3):e0033524. doi: 10.1128/mbio.00335-24. PMID: 38903064 Free PMC article. Updated. Preprint.
-
Coevolution of Eukaryote-like Vps4 and ESCRT-III Subunits in the Asgard Archaea.mBio. 2020 May 19;11(3):e00417-20. doi: 10.1128/mBio.00417-20. mBio. 2020. PMID: 32430468 Free PMC article.
-
Asgard archaea shed light on the evolutionary origins of the eukaryotic ubiquitin-ESCRT machinery.Nat Commun. 2022 Jun 13;13(1):3398. doi: 10.1038/s41467-022-30656-2. Nat Commun. 2022. PMID: 35697693 Free PMC article.
-
Evolution of diverse cell division and vesicle formation systems in Archaea.Nat Rev Microbiol. 2010 Oct;8(10):731-41. doi: 10.1038/nrmicro2406. Epub 2010 Sep 6. Nat Rev Microbiol. 2010. PMID: 20818414 Free PMC article. Review.
-
The Structure, Function and Roles of the Archaeal ESCRT Apparatus.Subcell Biochem. 2017;84:357-377. doi: 10.1007/978-3-319-53047-5_12. Subcell Biochem. 2017. PMID: 28500532 Review.
Cited by
-
Navigating the archaeal frontier: insights and projections from bioinformatic pipelines.Front Microbiol. 2024 Sep 23;15:1433224. doi: 10.3389/fmicb.2024.1433224. eCollection 2024. Front Microbiol. 2024. PMID: 39380680 Free PMC article.
-
Preserving Genome Integrity: Unveiling the Roles of ESCRT Machinery.Cells. 2024 Aug 5;13(15):1307. doi: 10.3390/cells13151307. Cells. 2024. PMID: 39120335 Free PMC article. Review.
References
MeSH terms
Substances
Grants and funding
LinkOut - more resources
Full Text Sources