Structural insights into peptidoglycan glycosidase EtgA binding to the inner rod protein EscI of the type III secretion system via a designed EscI-EtgA fusion protein
- PMID: 38380768
- PMCID: PMC10880428
- DOI: 10.1002/pro.4930
Structural insights into peptidoglycan glycosidase EtgA binding to the inner rod protein EscI of the type III secretion system via a designed EscI-EtgA fusion protein
Abstract
Bacteria express lytic enzymes such as glycosidases, which have potentially self-destructive peptidoglycan (PG)-degrading activity and, therefore, require careful regulation in bacteria. The PG glycosidase EtgA is regulated by localization to the assembling type III secretion system (T3SS), generating a hole in the PG layer for the T3SS to reach the outer membrane. The EtgA localization was found to be mediated via EtgA interacting with the T3SS inner rod protein EscI. To gain structural insights into the EtgA recognition of EscI, we determined the 2.01 Å resolution structure of an EscI (51-87)-linker-EtgA fusion protein designed based on AlphaFold2 predictions. The structure revealed EscI residues 72-87 forming an α-helix interacting with the backside of EtgA, distant from the active site. EscI residues 56-71 also were found to interact with EtgA, with these residues stretching across the EtgA surface. The ability of the EscI to interact with EtgA was also probed using an EscI peptide. The EscI peptide comprising residues 66-87, slightly larger than the observed EscI α-helix, was shown to bind to EtgA using microscale thermophoresis and thermal shift differential scanning fluorimetry. The EscI peptide also had a two-fold activity-enhancing effect on EtgA, whereas the EscI-EtgA fusion protein enhanced activity over four-fold compared to EtgA. Our studies suggest that EtgA regulation by EscI could be trifold involving protein localization, protein activation, and protein stabilization components. Analysis of the sequence conservation of the EscI EtgA interface residues suggested a possible conservation of such regulation for related proteins from different bacteria.
Keywords: EscI inner rod protein; lytic transglycosylase; muramidase; peptidoglycan glycosidases EtgA; protein crystallography; type III secretion system.
© 2024 The Authors. Protein Science published by Wiley Periodicals LLC on behalf of The Protein Society.
Figures
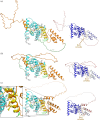
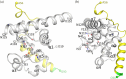
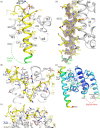
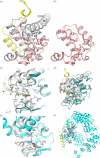
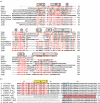
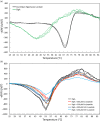
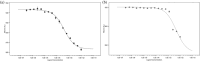
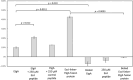
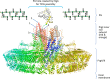
Similar articles
-
Structural analysis of a specialized type III secretion system peptidoglycan-cleaving enzyme.J Biol Chem. 2015 Apr 17;290(16):10406-17. doi: 10.1074/jbc.M115.639013. Epub 2015 Feb 12. J Biol Chem. 2015. PMID: 25678709 Free PMC article.
-
The muramidase EtgA from enteropathogenic Escherichia coli is required for efficient type III secretion.Microbiology (Reading). 2011 Apr;157(Pt 4):1145-1160. doi: 10.1099/mic.0.045617-0. Epub 2011 Jan 13. Microbiology (Reading). 2011. PMID: 21233160
-
Enhanced protein translocation to mammalian cells by expression of EtgA transglycosylase in a synthetic injector E. coli strain.Microb Cell Fact. 2022 Jul 2;21(1):133. doi: 10.1186/s12934-022-01860-y. Microb Cell Fact. 2022. PMID: 35780105 Free PMC article.
-
Masters of Misdirection: Peptidoglycan Glycosidases in Bacterial Growth.J Bacteriol. 2023 Mar 21;205(3):e0042822. doi: 10.1128/jb.00428-22. Epub 2023 Feb 9. J Bacteriol. 2023. PMID: 36757204 Free PMC article. Review.
-
Protein Export via the Type III Secretion System of the Bacterial Flagellum.Biomolecules. 2021 Jan 29;11(2):186. doi: 10.3390/biom11020186. Biomolecules. 2021. PMID: 33572887 Free PMC article. Review.
References
MeSH terms
Substances
Grants and funding
LinkOut - more resources
Full Text Sources