Chemoproteomics-based profiling reveals potential antimalarial mechanism of Celastrol by disrupting spermidine and protein synthesis
- PMID: 38378659
- PMCID: PMC10877925
- DOI: 10.1186/s12964-023-01409-5
Chemoproteomics-based profiling reveals potential antimalarial mechanism of Celastrol by disrupting spermidine and protein synthesis
Abstract
Background: Malaria remains a global health burden, and the emergence and increasing spread of drug resistance to current antimalarials poses a major challenge to malaria control. There is an urgent need to find new drugs or strategies to alleviate this predicament. Celastrol (Cel) is an extensively studied natural bioactive compound that has shown potentially promising antimalarial activity, but its antimalarial mechanism remains largely elusive.
Methods: We first established the Plasmodium berghei ANKA-infected C57BL/6 mouse model and systematically evaluated the antimalarial effects of Cel in conjunction with in vitro culture of Plasmodium falciparum. The potential antimalarial targets of Cel were then identified using a Cel activity probe based on the activity-based protein profiling (ABPP) technology. Subsequently, the antimalarial mechanism was analyzed by integrating with proteomics and transcriptomics. The binding of Cel to the identified key target proteins was verified by a series of biochemical experiments and functional assays.
Results: The results of the pharmacodynamic assay showed that Cel has favorable antimalarial activity both in vivo and in vitro. The ABPP-based target profiling showed that Cel can bind to a number of proteins in the parasite. Among the 31 identified potential target proteins of Cel, PfSpdsyn and PfEGF1-α were verified to be two critical target proteins, suggesting the role of Cel in interfering with the de novo synthesis of spermidine and proteins of the parasite, thus exerting its antimalarial effects.
Conclusions: In conclusion, this study reports for the first time the potential antimalarial targets and mechanism of action of Cel using the ABPP strategy. Our work not only support the expansion of Cel as a potential antimalarial agent or adjuvant, but also establishes the necessary theoretical basis for the development of potential antimalarial drugs with pentacyclic triterpenoid structures, as represented by Cel. Video Abstract.
Keywords: Antimalarial; Celastrol; Protein synthesis; Spermidine.
© 2024. The Author(s).
Conflict of interest statement
The authors declare no competing interests.
Figures
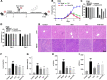
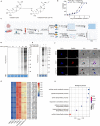
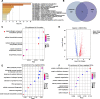
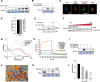
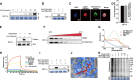
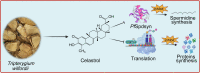
Similar articles
-
Folic acid supplementation and malaria susceptibility and severity among people taking antifolate antimalarial drugs in endemic areas.Cochrane Database Syst Rev. 2022 Feb 1;2(2022):CD014217. doi: 10.1002/14651858.CD014217. Cochrane Database Syst Rev. 2022. PMID: 36321557 Free PMC article.
-
Antimalarial efficacy of Pongamia pinnata (L) Pierre against Plasmodium falciparum (3D7 strain) and Plasmodium berghei (ANKA).BMC Complement Altern Med. 2017 Sep 11;17(1):458. doi: 10.1186/s12906-017-1958-y. BMC Complement Altern Med. 2017. Retraction in: BMC Complement Med Ther. 2021 May 10;21(1):139. doi: 10.1186/s12906-021-03312-3. PMID: 28893216 Free PMC article. Retracted.
-
Protective activity of biflavanones from Garcinia kola against Plasmodium infection.J Ethnopharmacol. 2015 Aug 22;172:214-8. doi: 10.1016/j.jep.2015.06.038. Epub 2015 Jun 27. J Ethnopharmacol. 2015. PMID: 26129936
-
Chemoproteomics for Plasmodium Parasite Drug Target Discovery.Chembiochem. 2021 Aug 17;22(16):2591-2599. doi: 10.1002/cbic.202100155. Epub 2021 Jun 10. Chembiochem. 2021. PMID: 33999499 Free PMC article. Review.
-
[Progress of researches on antimalarial peptides].Zhongguo Xue Xi Chong Bing Fang Zhi Za Zhi. 2023 Apr 19;35(2):191-198. doi: 10.16250/j.32.1374.2023011. Zhongguo Xue Xi Chong Bing Fang Zhi Za Zhi. 2023. PMID: 37253570 Review. Chinese.
References
-
- WHO . World malaria report 2022 [internet] Geneva, Switzerland: WHO; 2022.
Publication types
MeSH terms
Substances
LinkOut - more resources
Full Text Sources
Medical