Synergistic Protection of Retinal Ganglion Cells (RGCs) by SARM1 Inactivation with CNTF in a Rodent Model of Nonarteritic Anterior Ischemic Optic Neuropathy
- PMID: 38334594
- PMCID: PMC10854792
- DOI: 10.3390/cells13030202
Synergistic Protection of Retinal Ganglion Cells (RGCs) by SARM1 Inactivation with CNTF in a Rodent Model of Nonarteritic Anterior Ischemic Optic Neuropathy
Abstract
We evaluated whether inhibiting sterile alpha and (Toll/interleukin receptor (TIR)) motif-containing 1 (SARM1) activity protects retinal ganglion cells (RGCs) following ischemic axonopathy (rodent nonarteritic anterior ischemic optic neuropathy: rNAION) by itself and combined with ciliary neurotrophic factor (CNTF). Genetically modified SARM1(-) rats were rNAION-induced in one eye and compared against equivalently induced wild-type animals of the same background. Optic nerve (ON) diameters were quantified using optical coherence tomography (SD-OCT). RGCs were quantified 30 d post-induction using retinal stereology for Brn3a(+) nuclei. ON sections were analyzed by TEM and immunohistochemistry. SARM1(-)(-) and WT animals were then bilaterally sequentially rNAION-induced. One eye received intravitreal vehicle injection following induction; the contralateral side received CNTF and was analyzed 30 d post-induction. Inhibiting SARM1 activity suppressed axonal collapse following ischemic axonopathy. SARM1(-) animals significantly reduced RGC loss, compared with WT animals (49.4 ± 6.8% RGC loss in SARM1(-) vs. 63.6 ± 3.2% sem RGC loss in WT; Mann-Whitney one-tailed U-test, (p = 0.049)). IVT-CNTF treatment vs. IVT-vehicle in SARM1(-) animals further reduced RGC loss by 24% at 30 d post-induction, but CNTF did not, by itself, improve long-term RGC survival in WT animals compared with vehicle (Mann-Whitney one-tailed t-test; p = 0.033). While inhibiting SARM1 activity is itself neuroprotective, combining SARM1 inhibition and CNTF treatment generated a long-term, synergistic neuroprotective effect in ischemic neuropathy. Combinatorial treatments for NAION utilizing independent neuroprotective mechanisms may thus provide a greater effect than individual treatment modalities.
Keywords: axonopathy; ciliary neurotrophic factor; ischemia; neuroprotection; nonarteritic anterior ischemic optic neuropathy (NAION); optic nerve; retinal ganglion cells; rodent; sterile alpha and (toll/interleukin receptor (TIR)) motif-containing 1 (SARM1); synergism.
Conflict of interest statement
The authors declare no conflicts of interest.
Figures
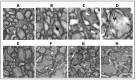
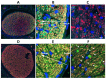
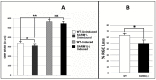
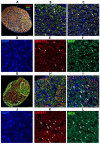
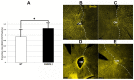
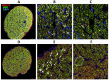
Similar articles
-
Intravitreal AAV2 gene delivery to feline retinal ganglion cells.Vision Res. 2025 Jan;226:108519. doi: 10.1016/j.visres.2024.108519. Epub 2024 Nov 16. Vision Res. 2025. PMID: 39549467 Free PMC article.
-
Remote Ischemic Post-Conditioning (RIC) Mediates Anti-Inflammatory Signaling via Myeloid AMPKα1 in Murine Traumatic Optic Neuropathy (TON).Int J Mol Sci. 2024 Dec 19;25(24):13626. doi: 10.3390/ijms252413626. Int J Mol Sci. 2024. PMID: 39769388 Free PMC article.
-
Regulation of disease-associated microglia in the optic nerve by lipoxin B4 and ocular hypertension.Mol Neurodegener. 2024 Nov 20;19(1):86. doi: 10.1186/s13024-024-00775-z. Mol Neurodegener. 2024. PMID: 39568070 Free PMC article.
-
[Nonarteritic ischemic optic neuropathy animal model and its treatment applications].Nippon Ganka Gakkai Zasshi. 2014 Apr;118(4):331-61. Nippon Ganka Gakkai Zasshi. 2014. PMID: 24864434 Review. Japanese.
-
Retinal ganglion cell repopulation for vision restoration in optic neuropathy: a roadmap from the RReSTORe Consortium.Mol Neurodegener. 2023 Sep 21;18(1):64. doi: 10.1186/s13024-023-00655-y. Mol Neurodegener. 2023. PMID: 37735444 Free PMC article. Review.
References
-
- Chan C.K., Cheng A.C., Leung C.K., Cheung C.Y., Yung A.Y., Gong B., Lam D.S. Quantitative assessment of optic nerve head morphology and retinal nerve fibre layer in non-arteritic anterior ischaemic optic neuropathy with optical coherence tomography and confocal scanning laser ophthalmoloscopy. Br. J. Ophthalmol. 2009;93:731–735. doi: 10.1136/bjo.2008.143297. - DOI - PubMed
Publication types
MeSH terms
Substances
Grants and funding
LinkOut - more resources
Full Text Sources
Miscellaneous