This is a preprint.
MagIC-Cryo-EM: Structural determination on magnetic beads for scarce macromolecules in heterogeneous samples
- PMID: 38328033
- PMCID: PMC10849486
- DOI: 10.1101/2024.01.21.576499
MagIC-Cryo-EM: Structural determination on magnetic beads for scarce macromolecules in heterogeneous samples
Abstract
Cryo-EM single-particle analyses typically require target macromolecule concentration at 0.05~5.0 mg/ml, which is often difficult to achieve. Here, we devise Magnetic Isolation and Concentration (MagIC)-cryo-EM, a technique enabling direct structural analysis of targets captured on magnetic beads, thereby reducing the targets' concentration requirement to < 0.0005 mg/ml. Adapting MagIC-cryo-EM to a Chromatin Immunoprecipitation protocol, we characterized structural variations of the linker histone H1.8-associated nucleosomes that were isolated from interphase and metaphase chromosomes in Xenopus egg extract. Combining Duplicated Selection To Exclude Rubbish particles (DuSTER), a particle curation method that excludes low signal-to-noise ratio particles, we also resolved the 3D cryo-EM structures of nucleoplasmin NPM2 co-isolated with the linker histone H1.8 and revealed distinct open and closed structural variants. Our study demonstrates the utility of MagIC-cryo-EM for structural analysis of scarce macromolecules in heterogeneous samples and provides structural insights into the cell cycle-regulation of H1.8 association to nucleosomes.
Conflict of interest statement
Declaration of interests YA, HAK, and HF have filed a patent application encompassing aspects of MagIC-cryo-EM (PCT/US2023/03315). HF is affiliated with the Graduate School of Medical Sciences, Weill Cornell Medicine, and Cell Biology Program at the Sloan Kettering Institute.
Figures
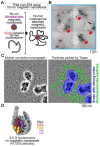
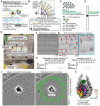
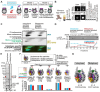
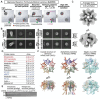
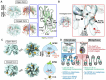
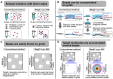
Similar articles
-
Erratum: Eyestalk Ablation to Increase Ovarian Maturation in Mud Crabs.J Vis Exp. 2023 May 26;(195). doi: 10.3791/6561. J Vis Exp. 2023. PMID: 37235796
-
[Construction of a 17β-estradiol sensor based on a magnetic graphene oxide/aptamer separating material].Se Pu. 2025 Jan;43(1):87-95. doi: 10.3724/SP.J.1123.2024.06009. Se Pu. 2025. PMID: 39722625 Free PMC article. Chinese.
-
Structure and identification of the native PLP synthase complex from Methanosarcina acetivorans lysate.mBio. 2025 Jan 8;16(1):e0309024. doi: 10.1128/mbio.03090-24. Epub 2024 Nov 26. mBio. 2025. PMID: 39589128 Free PMC article.
-
Depressing time: Waiting, melancholia, and the psychoanalytic practice of care.In: Kirtsoglou E, Simpson B, editors. The Time of Anthropology: Studies of Contemporary Chronopolitics. Abingdon: Routledge; 2020. Chapter 5. In: Kirtsoglou E, Simpson B, editors. The Time of Anthropology: Studies of Contemporary Chronopolitics. Abingdon: Routledge; 2020. Chapter 5. PMID: 36137063 Free Books & Documents. Review.
-
Intratympanic corticosteroids for Ménière's disease.Cochrane Database Syst Rev. 2023 Feb 27;2(2):CD015245. doi: 10.1002/14651858.CD015245.pub2. Cochrane Database Syst Rev. 2023. PMID: 36847608 Free PMC article. Review.
References
-
- Leesch F., Lorenzo-Orts L., Pribitzer C., Grishkovskaya I., Roehsner J., Chugunova A., Matzinger M., Roitinger E., Belačić K., Kandolf S., et al. (2023). A molecular network of conserved factors keeps ribosomes dormant in the egg. Nature 613, 712–720. 10.1038/s41586-022-05623-y. - DOI - PMC - PubMed
Publication types
Grants and funding
LinkOut - more resources
Full Text Sources
Research Materials