Arabidopsis COP1 guides stomatal response in guard cells through pH regulation
- PMID: 38316905
- PMCID: PMC10844630
- DOI: 10.1038/s42003-024-05847-w
Arabidopsis COP1 guides stomatal response in guard cells through pH regulation
Abstract
Plants rely on precise regulation of their stomatal pores to effectively carry out photosynthesis while managing water status. The Arabidopsis CONSTITUTIVE PHOTOMORPHOGENIC 1 (COP1), a critical light signaling repressor, is known to repress stomatal opening, but the exact cellular mechanisms remain unknown. Here, we show that COP1 regulates stomatal movement by controlling the pH levels in guard cells. cop1-4 mutants have larger stomatal apertures and disrupted pH dynamics within guard cells, characterized by increased vacuolar and cytosolic pH and reduced apoplastic pH, leading to abnormal stomatal responses. The altered pH profiles are attributed to the increased plasma membrane (PM) H+-ATPase activity of cop1-4 mutants. Moreover, cop1-4 mutants resist to growth defect caused by alkali stress posed on roots. Overall, our study highlights the crucial role of COP1 in maintaining pH homeostasis of guard cells by regulating PM H+-ATPase activity, and demonstrates how proton movement affects stomatal movement and plant growth.
© 2024. The Author(s).
Conflict of interest statement
The authors declare no competing interests.
Figures
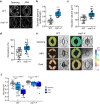
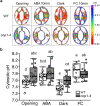
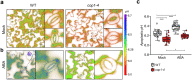
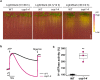
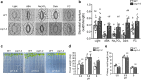
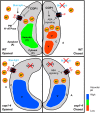
Similar articles
-
Mitochondrial ß-Cyanoalanine Synthase Participates in flg22-Induced Stomatal Immunity.Plant Cell Environ. 2025 Jan;48(1):537-552. doi: 10.1111/pce.15155. Epub 2024 Sep 17. Plant Cell Environ. 2025. PMID: 39288437
-
An Arabidopsis Plasma Membrane Proton ATPase Modulates JA Signaling and Is Exploited by the Pseudomonas syringae Effector Protein AvrB for Stomatal Invasion.Plant Cell. 2015 Jul;27(7):2032-41. doi: 10.1105/tpc.15.00466. Epub 2015 Jul 21. Plant Cell. 2015. PMID: 26198069 Free PMC article.
-
Using Experience Sampling Methodology to Capture Disclosure Opportunities for Autistic Adults.Autism Adulthood. 2023 Dec 1;5(4):389-400. doi: 10.1089/aut.2022.0090. Epub 2023 Dec 12. Autism Adulthood. 2023. PMID: 38116059 Free PMC article.
-
Depressing time: Waiting, melancholia, and the psychoanalytic practice of care.In: Kirtsoglou E, Simpson B, editors. The Time of Anthropology: Studies of Contemporary Chronopolitics. Abingdon: Routledge; 2020. Chapter 5. In: Kirtsoglou E, Simpson B, editors. The Time of Anthropology: Studies of Contemporary Chronopolitics. Abingdon: Routledge; 2020. Chapter 5. PMID: 36137063 Free Books & Documents. Review.
-
Pharmacological treatments in panic disorder in adults: a network meta-analysis.Cochrane Database Syst Rev. 2023 Nov 28;11(11):CD012729. doi: 10.1002/14651858.CD012729.pub3. Cochrane Database Syst Rev. 2023. PMID: 38014714 Free PMC article. Review.
Cited by
-
Cytosolic alkalinization in guard cells: an intriguing but interesting event during stomatal closure that merits further validation of its importance.Front Plant Sci. 2024 Nov 4;15:1491428. doi: 10.3389/fpls.2024.1491428. eCollection 2024. Front Plant Sci. 2024. PMID: 39559765 Free PMC article. Review.
References
Publication types
MeSH terms
Substances
LinkOut - more resources
Full Text Sources