Multi-modal cryo-EM reveals trimers of protein A10 to form the palisade layer in poxvirus cores
- PMID: 38316877
- PMCID: PMC11257981
- DOI: 10.1038/s41594-023-01201-6
Multi-modal cryo-EM reveals trimers of protein A10 to form the palisade layer in poxvirus cores
Abstract
Poxviruses are among the largest double-stranded DNA viruses, with members such as variola virus, monkeypox virus and the vaccination strain vaccinia virus (VACV). Knowledge about the structural proteins that form the viral core has remained sparse. While major core proteins have been annotated via indirect experimental evidence, their structures have remained elusive and they could not be assigned to individual core features. Hence, which proteins constitute which layers of the core, such as the palisade layer and the inner core wall, has remained enigmatic. Here we show, using a multi-modal cryo-electron microscopy (cryo-EM) approach in combination with AlphaFold molecular modeling, that trimers formed by the cleavage product of VACV protein A10 are the key component of the palisade layer. This allows us to place previously obtained descriptions of protein interactions within the core wall into perspective and to provide a detailed model of poxvirus core architecture. Importantly, we show that interactions within A10 trimers are likely generalizable over members of orthopox- and parapoxviruses.
© 2024. The Author(s).
Conflict of interest statement
The authors have no competing interests.
Figures
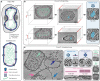
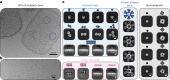
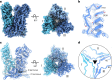
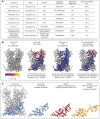
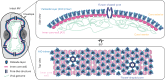
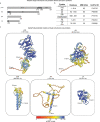
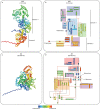
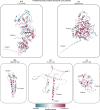
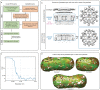
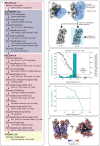
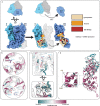
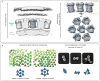
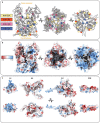
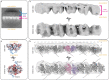
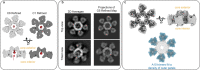
Similar articles
-
Depressing time: Waiting, melancholia, and the psychoanalytic practice of care.In: Kirtsoglou E, Simpson B, editors. The Time of Anthropology: Studies of Contemporary Chronopolitics. Abingdon: Routledge; 2020. Chapter 5. In: Kirtsoglou E, Simpson B, editors. The Time of Anthropology: Studies of Contemporary Chronopolitics. Abingdon: Routledge; 2020. Chapter 5. PMID: 36137063 Free Books & Documents. Review.
-
Qualitative evidence synthesis informing our understanding of people's perceptions and experiences of targeted digital communication.Cochrane Database Syst Rev. 2019 Oct 23;10(10):ED000141. doi: 10.1002/14651858.ED000141. Cochrane Database Syst Rev. 2019. PMID: 31643081 Free PMC article.
-
Unlocking data: Decision-maker perspectives on cross-sectoral data sharing and linkage as part of a whole-systems approach to public health policy and practice.Public Health Res (Southampt). 2024 Nov 20:1-30. doi: 10.3310/KYTW2173. Online ahead of print. Public Health Res (Southampt). 2024. PMID: 39582242
-
Defining the optimum strategy for identifying adults and children with coeliac disease: systematic review and economic modelling.Health Technol Assess. 2022 Oct;26(44):1-310. doi: 10.3310/ZUCE8371. Health Technol Assess. 2022. PMID: 36321689 Free PMC article.
-
Trends in Surgical and Nonsurgical Aesthetic Procedures: A 14-Year Analysis of the International Society of Aesthetic Plastic Surgery-ISAPS.Aesthetic Plast Surg. 2024 Oct;48(20):4217-4227. doi: 10.1007/s00266-024-04260-2. Epub 2024 Aug 5. Aesthetic Plast Surg. 2024. PMID: 39103642 Review.
Cited by
-
Palisade structure in intact vaccinia virions.mBio. 2024 Feb 14;15(2):e0313423. doi: 10.1128/mbio.03134-23. Epub 2024 Jan 3. mBio. 2024. PMID: 38171004 Free PMC article.
-
Structure of the poxvirus core.Nat Struct Mol Biol. 2024 Jul;31(7):1001-1003. doi: 10.1038/s41594-024-01331-5. Nat Struct Mol Biol. 2024. PMID: 38890551 No abstract available.
-
Molecular architecture of monkeypox mature virus.Cell Discov. 2024 Oct 22;10(1):108. doi: 10.1038/s41421-024-00741-5. Cell Discov. 2024. PMID: 39438447 Free PMC article. No abstract available.
References
MeSH terms
Substances
Grants and funding
LinkOut - more resources
Full Text Sources