This is a preprint.
Divergent opioid-mediated suppression of inhibition between hippocampus and neocortex across species and development
- PMID: 38313283
- PMCID: PMC10836073
- DOI: 10.1101/2024.01.20.576455
Divergent opioid-mediated suppression of inhibition between hippocampus and neocortex across species and development
Abstract
Within the adult rodent hippocampus, opioids suppress inhibitory parvalbumin-expressing interneurons (PV-INs), thus disinhibiting local micro-circuits. However, it is unknown if this disinhibitory motif is conserved in other cortical regions, species, or across development. We observed that PV-IN mediated inhibition is robustly suppressed by opioids in hippocampus proper but not primary neocortex in mice and nonhuman primates, with spontaneous inhibitory tone in resected human tissue also following a consistent dichotomy. This hippocampal disinhibitory motif was established in early development when PV-INs and opioids were found to regulate early population activity. Acute opioid-mediated modulation was partially occluded with morphine pretreatment, with implications for the effects of opioids on hippocampal network activity important for learning and memory. Together, these findings demonstrate that PV-INs exhibit a divergence in opioid sensitivity across brain regions that is remarkably conserved across evolution and highlights the underappreciated role of opioids acting through immature PV-INs in shaping hippocampal development.
Conflict of interest statement
DECLARATION OF INTERESTS The authors declare no competing interests.
Figures
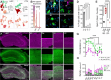
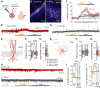
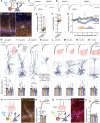
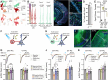
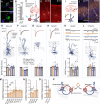
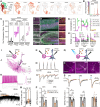
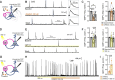
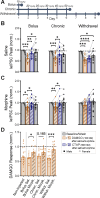
Similar articles
-
Depressing time: Waiting, melancholia, and the psychoanalytic practice of care.In: Kirtsoglou E, Simpson B, editors. The Time of Anthropology: Studies of Contemporary Chronopolitics. Abingdon: Routledge; 2020. Chapter 5. In: Kirtsoglou E, Simpson B, editors. The Time of Anthropology: Studies of Contemporary Chronopolitics. Abingdon: Routledge; 2020. Chapter 5. PMID: 36137063 Free Books & Documents. Review.
-
Using Experience Sampling Methodology to Capture Disclosure Opportunities for Autistic Adults.Autism Adulthood. 2023 Dec 1;5(4):389-400. doi: 10.1089/aut.2022.0090. Epub 2023 Dec 12. Autism Adulthood. 2023. PMID: 38116059 Free PMC article.
-
Ceftazidime with avibactam for treating severe aerobic Gram-negative bacterial infections: technology evaluation to inform a novel subscription-style payment model.Health Technol Assess. 2024 Oct;28(73):1-230. doi: 10.3310/YAPL9347. Health Technol Assess. 2024. PMID: 39487661 Free PMC article.
-
A Blog-Based Study of Autistic Adults' Experiences of Aloneness and Connection and the Interplay with Well-Being: Corpus-Based and Thematic Analyses.Autism Adulthood. 2023 Dec 1;5(4):437-449. doi: 10.1089/aut.2022.0073. Epub 2023 Dec 12. Autism Adulthood. 2023. PMID: 38116056 Free PMC article.
-
Metformin for endometrial hyperplasia.Cochrane Database Syst Rev. 2024 May 2;5(5):CD012214. doi: 10.1002/14651858.CD012214.pub3. Cochrane Database Syst Rev. 2024. PMID: 38695827 Review.
References
Publication types
Grants and funding
LinkOut - more resources
Full Text Sources