Phosphorylation of AQP4 by LRRK2 R1441G impairs glymphatic clearance of IFNγ and aggravates dopaminergic neurodegeneration
- PMID: 38296953
- PMCID: PMC10831045
- DOI: 10.1038/s41531-024-00643-z
Phosphorylation of AQP4 by LRRK2 R1441G impairs glymphatic clearance of IFNγ and aggravates dopaminergic neurodegeneration
Abstract
Aquaporin-4 (AQP4) is essential for normal functioning of the brain's glymphatic system. Impaired glymphatic function is associated with neuroinflammation. Recent clinical evidence suggests the involvement of glymphatic dysfunction in LRRK2-associated Parkinson's disease (PD); however, the precise mechanism remains unclear. The pro-inflammatory cytokine interferon (IFN) γ interacts with LRRK2 to induce neuroinflammation. Therefore, we examined the AQP4-dependent glymphatic system's role in IFNγ-mediated neuroinflammation in LRRK2-associated PD. We found that LRRK2 interacts with and phosphorylates AQP4 in vitro and in vivo. AQP4 phosphorylation by LRRK2 R1441G induced AQP4 depolarization and disrupted glymphatic IFNγ clearance. Exogeneous IFNγ significantly increased astrocyte expression of IFNγ receptor, amplified AQP4 depolarization, and exacerbated neuroinflammation in R1441G transgenic mice. Conversely, inhibiting LRRK2 restored AQP4 polarity, improved glymphatic function, and reduced IFNγ-mediated neuroinflammation and dopaminergic neurodegeneration. Our findings establish a link between LRRK2-mediated AQP4 phosphorylation and IFNγ-mediated neuroinflammation in LRRK2-associated PD, guiding the development of LRRK2 targeting therapy.
© 2024. The Author(s).
Conflict of interest statement
The authors declare no competing interests.
Figures
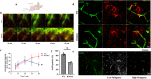
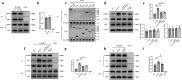
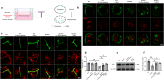
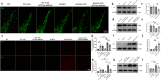
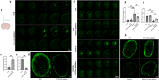
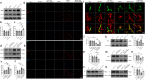
References
Grants and funding
LinkOut - more resources
Full Text Sources