This is a preprint.
Loss of dihydroceramide desaturase drives neurodegeneration by disrupting endoplasmic reticulum and lipid droplet homeostasis in glial cells
- PMID: 38260379
- PMCID: PMC10802327
- DOI: 10.1101/2024.01.01.573836
Loss of dihydroceramide desaturase drives neurodegeneration by disrupting endoplasmic reticulum and lipid droplet homeostasis in glial cells
Abstract
Dihydroceramide desaturases convert dihydroceramides to ceramides, the precursors of all complex sphingolipids. Reduction of DEGS1 dihydroceramide desaturase function causes pediatric neurodegenerative disorder hypomyelinating leukodystrophy-18 (HLD-18). We discovered that infertile crescent (ifc), the Drosophila DEGS1 homolog, is expressed primarily in glial cells to promote CNS development by guarding against neurodegeneration. Loss of ifc causes massive dihydroceramide accumulation and severe morphological defects in cortex glia, including endoplasmic reticulum (ER) expansion, failure of neuronal ensheathment, and lipid droplet depletion. RNAi knockdown of the upstream ceramide synthase schlank in glia of ifc mutants rescues ER expansion, suggesting dihydroceramide accumulation in the ER drives this phenotype. RNAi knockdown of ifc in glia but not neurons drives neuronal cell death, suggesting that ifc function in glia promotes neuronal survival. Our work identifies glia as the primary site of disease progression in HLD-18 and may inform on juvenile forms of ALS, which also feature elevated dihydroceramide levels.
Keywords: DEGS1; ceramide; endoplasmic reticulum; glia; leukodystrophy; lipid droplet; neurodegeneration; sphingolipids.
Conflict of interest statement
Declaration of interests: G.J.P. has collaborative research agreements with Agilent Technologies and Thermo Scientific. G.J.P. is the chief scientific officer of Panome Bio. The remaining authors declare no competing interests.
Figures
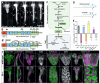
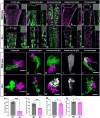
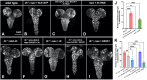
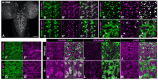
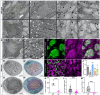
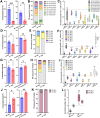
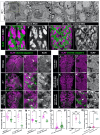
Similar articles
-
Dihydroceramide desaturase regulates the compartmentalization of Rac1 for neuronal oxidative stress.Cell Rep. 2021 Apr 13;35(2):108972. doi: 10.1016/j.celrep.2021.108972. Cell Rep. 2021. PMID: 33852856
-
Lipophagy prevents activity-dependent neurodegeneration due to dihydroceramide accumulation in vivo.EMBO Rep. 2017 Jul;18(7):1150-1165. doi: 10.15252/embr.201643480. Epub 2017 May 15. EMBO Rep. 2017. PMID: 28507162 Free PMC article.
-
Dihydroceramide desaturase promotes the formation of intraluminal vesicles and inhibits autophagy to increase exosome production.iScience. 2021 Nov 14;24(12):103437. doi: 10.1016/j.isci.2021.103437. eCollection 2021 Dec 17. iScience. 2021. PMID: 34877496 Free PMC article.
-
The pathophysiological role of dihydroceramide desaturase in the nervous system.Prog Lipid Res. 2023 Jul;91:101236. doi: 10.1016/j.plipres.2023.101236. Epub 2023 May 13. Prog Lipid Res. 2023. PMID: 37187315 Review.
-
DEGS1 -related leukodystrophy: a clinical report and review of literature.Clin Dysmorphol. 2023 Jul 1;32(3):106-111. doi: 10.1097/MCD.0000000000000457. Epub 2023 May 1. Clin Dysmorphol. 2023. PMID: 37195341 Review.
References
Publication types
Grants and funding
LinkOut - more resources
Full Text Sources
Miscellaneous