This is a preprint.
Impaired biogenesis of basic proteins impacts multiple hallmarks of the aging brain
- PMID: 38260253
- PMCID: PMC10802395
- DOI: 10.1101/2023.07.20.549210
Impaired biogenesis of basic proteins impacts multiple hallmarks of the aging brain
Abstract
Aging and neurodegeneration entail diverse cellular and molecular hallmarks. Here, we studied the effects of aging on the transcriptome, translatome, and multiple layers of the proteome in the brain of a short-lived killifish. We reveal that aging causes widespread reduction of proteins enriched in basic amino acids that is independent of mRNA regulation, and it is not due to impaired proteasome activity. Instead, we identify a cascade of events where aberrant translation pausing leads to reduced ribosome availability resulting in proteome remodeling independently of transcriptional regulation. Our research uncovers a vulnerable point in the aging brain's biology - the biogenesis of basic DNA/RNA binding proteins. This vulnerability may represent a unifying principle that connects various aging hallmarks, encompassing genome integrity and the biosynthesis of macromolecules.
Keywords: aging; brain; killifish; mitochondria; post-translational modification; proteasome; protein aggregation; proteome; ribosome; translation.
Conflict of interest statement
Declaration of interest Authors declare no competing interests.
Figures
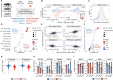
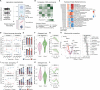
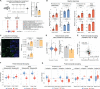
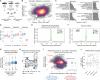
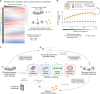
Similar articles
-
Reduced proteasome activity in the aging brain results in ribosome stoichiometry loss and aggregation.Mol Syst Biol. 2020 Jun;16(6):e9596. doi: 10.15252/msb.20209596. Mol Syst Biol. 2020. PMID: 32558274 Free PMC article.
-
Extensive Translational Regulation through the Proliferative Transition of Trypanosoma cruzi Revealed by Multi-Omics.mSphere. 2021 Oct 27;6(5):e0036621. doi: 10.1128/mSphere.00366-21. Epub 2021 Sep 1. mSphere. 2021. PMID: 34468164 Free PMC article.
-
Turquoise killifish: A natural model of age-dependent brain degeneration.Ageing Res Rev. 2023 Sep;90:102019. doi: 10.1016/j.arr.2023.102019. Epub 2023 Jul 22. Ageing Res Rev. 2023. PMID: 37482345 Review.
-
Alternative Polyadenylation Utilization Results in Ribosome Assembly and mRNA Translation Deficiencies in a Model for Muscle Aging.J Gerontol A Biol Sci Med Sci. 2022 Jun 1;77(6):1130-1140. doi: 10.1093/gerona/glac058. J Gerontol A Biol Sci Med Sci. 2022. PMID: 35245938 Free PMC article.
-
Translational control in aging and neurodegeneration.Wiley Interdiscip Rev RNA. 2021 Jul;12(4):e1628. doi: 10.1002/wrna.1628. Epub 2020 Sep 20. Wiley Interdiscip Rev RNA. 2021. PMID: 32954679 Free PMC article. Review.
References
-
- Labbadia J., Morimoto R. I., The biology of proteostasis in aging and disease. Annu. Rev. Biochem. (2015) (available at https://www.annualreviews.org/doi/abs/10.1146/annurev-biochem-060614-033955). - DOI - PMC - PubMed
-
- Hipp M. S., Kasturi P., Hartl F. U., The proteostasis network and its decline in ageing. Nat. Rev. Mol. Cell Biol. 20, 421–435 (2019). - PubMed
-
- Valenzano D. R., Terzibasi E., Cattaneo A., Domenici L., Cellerino A., Temperature affects longevity and age-related locomotor and cognitive decay in the short-lived fish Nothobranchius furzeri. Aging Cell. 5, 275–278 (2006). - PubMed
-
- Matsui H., Kenmochi N., Namikawa K., Age- and α-Synuclein-Dependent Degeneration of Dopamine and Noradrenaline Neurons in the Annual Killifish Nothobranchius furzeri. Cell Rep. 26, 1727–1733.e6 (2019). - PubMed
Publication types
Grants and funding
LinkOut - more resources
Full Text Sources