Preparation and Characterization of Acrylic and Methacrylic Phospholipid-Mimetic Polymer Hydrogels and Their Applications in Optical Tissue Clearing
- PMID: 38257040
- PMCID: PMC10820725
- DOI: 10.3390/polym16020241
Preparation and Characterization of Acrylic and Methacrylic Phospholipid-Mimetic Polymer Hydrogels and Their Applications in Optical Tissue Clearing
Abstract
The 2-methacryloyloxyethyl phosphorylcholine (MPC) polymers are mimetic to phospholipids, being widely used as biocompatible polymers. In our previous study, MPC polymer hydrogels proved more effective for optical tissue clearing compared to acrylamide (AAm) polymer hydrogels. In the present study, 2-acryloyloxyethyl phosphorylcholine (APC) was synthesized and employed to create hydrogels for a comparative analysis with methacrylic MPC-based hydrogels. APC, an acrylic monomer, was copolymerized with AAm in a similar reactivity. In contrast, MPC, as a methacrylic monomer, demonstrated higher copolymerization reactivity than AAm, leading to a spontaneously delayed two-step polymerization behavior. This suggests that the polymer sequences and network structures became heterogeneous when both methacrylic and acrylic monomers, as well as crosslinkers, were present in the copolymerization system. The molecular weight of the APC polymers was considerably smaller than that of the MPC polymers due to the formation of mid-chain radicals and subsequent β-scission during polymerization. The swelling ratios in water and strain sweep profiles of hydrogels prepared using acrylic and methacrylic compounds differed from those of hydrogels prepared using only acrylic compounds. This implies that copolymerization reactivity influences the polymer network structures and crosslinking density in addition to the copolymer composition. APC-based hydrogels are effective for the optical clearing of tumor tissues and are applicable to both passive and electrophoretic methods.
Keywords: copolymerization; hydrogel; optical tissue clearing; phosphorylcholine; viscoelasticity.
Conflict of interest statement
The authors declare no conflicts of interest.
Figures

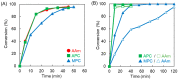
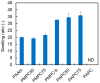
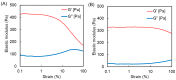
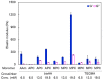
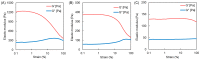
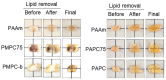
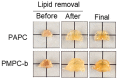
Similar articles
-
Application of Zwitterionic Polymer Hydrogels to Optical Tissue Clearing for 3D Fluorescence Imaging.Macromol Biosci. 2021 Sep;21(9):e2100170. doi: 10.1002/mabi.202100170. Epub 2021 Jun 21. Macromol Biosci. 2021. PMID: 34155811
-
Biocompatibility and drug release behavior of spontaneously formed phospholipid polymer hydrogels.J Biomed Mater Res A. 2007 Jan;80(1):45-54. doi: 10.1002/jbm.a.30864. J Biomed Mater Res A. 2007. PMID: 16958047
-
Spontaneously forming hydrogel from water-soluble random- and block-type phospholipid polymers.Biomaterials. 2005 Dec;26(34):6853-62. doi: 10.1016/j.biomaterials.2005.05.018. Biomaterials. 2005. PMID: 15978662
-
[Design of Intelligent Interface Based on Cytocompatible Polymers for Control on Cell Function].Yakugaku Zasshi. 2021;141(5):641-646. doi: 10.1248/yakushi.20-00219-3. Yakugaku Zasshi. 2021. PMID: 33952745 Review. Japanese.
-
Copolymers of 2-methacryloyloxyethyl phosphorylcholine (MPC) as biomaterials.Biomed Mater Eng. 2004;14(4):345-54. Biomed Mater Eng. 2004. PMID: 15472384 Review.
References
-
- Lau S.K., Yong W.F. Recent progress of zwitterionic materials as antifouling membranes for ultrafiltration, nanofiltration, and reverse osmosis. ACS Appl. Polym. Mater. 2021;3:4390–4412. doi: 10.1021/acsapm.1c00779. - DOI
Grants and funding
LinkOut - more resources
Full Text Sources