NAC and Zuotin/Hsp70 chaperone systems coexist at the ribosome tunnel exit in vivo
- PMID: 38224454
- PMCID: PMC11014269
- DOI: 10.1093/nar/gkae005
NAC and Zuotin/Hsp70 chaperone systems coexist at the ribosome tunnel exit in vivo
Abstract
The area surrounding the tunnel exit of the 60S ribosomal subunit is a hub for proteins involved in maturation and folding of emerging nascent polypeptide chains. How different factors vie for positioning at the tunnel exit in the complex cellular environment is not well understood. We used in vivo site-specific cross-linking to approach this question, focusing on two abundant factors-the nascent chain-associated complex (NAC) and the Hsp70 chaperone system that includes the J-domain protein co-chaperone Zuotin. We found that NAC and Zuotin can cross-link to each other at the ribosome, even when translation initiation is inhibited. Positions yielding NAC-Zuotin cross-links indicate that when both are present the central globular domain of NAC is modestly shifted from the mutually exclusive position observed in cryogenic electron microscopy analysis. Cross-linking results also suggest that, even in NAC's presence, Hsp70 can situate in a manner conducive for productive nascent chain interaction-with the peptide binding site at the tunnel exit and the J-domain of Zuotin appropriately positioned to drive stabilization of nascent chain binding. Overall, our results are consistent with the idea that, in vivo, the NAC and Hsp70 systems can productively position on the ribosome simultaneously.
© The Author(s) 2024. Published by Oxford University Press on behalf of Nucleic Acids Research.
Figures
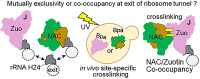
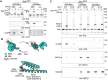
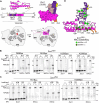
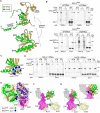

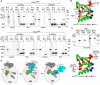
Similar articles
-
Structure and evolution of the 4-helix bundle domain of Zuotin, a J-domain protein co-chaperone of Hsp70.PLoS One. 2019 May 15;14(5):e0217098. doi: 10.1371/journal.pone.0217098. eCollection 2019. PLoS One. 2019. PMID: 31091298 Free PMC article.
-
A conserved motif is prerequisite for the interaction of NAC with ribosomal protein L23 and nascent chains.J Biol Chem. 2006 Feb 3;281(5):2847-57. doi: 10.1074/jbc.M511420200. Epub 2005 Nov 29. J Biol Chem. 2006. PMID: 16316984
-
Pathway of Hsp70 interactions at the ribosome.Nat Commun. 2021 Sep 27;12(1):5666. doi: 10.1038/s41467-021-25930-8. Nat Commun. 2021. PMID: 34580293 Free PMC article.
-
Folding of a nascent peptide on the ribosome.Prog Nucleic Acid Res Mol Biol. 2001;66:41-66. doi: 10.1016/s0079-6603(00)66026-9. Prog Nucleic Acid Res Mol Biol. 2001. PMID: 11051761 Review.
-
The ribosome-bound Hsp70 homolog Ssb of Saccharomyces cerevisiae.Biochim Biophys Acta. 2010 Jun;1803(6):662-72. doi: 10.1016/j.bbamcr.2010.03.005. Epub 2010 Mar 11. Biochim Biophys Acta. 2010. PMID: 20226819 Review.
Cited by
-
Functional similarities and differences among subunits of the nascent polypeptide-associated complex (NAC) of Saccharomyces cerevisiae.Cell Stress Chaperones. 2024 Dec;29(6):721-734. doi: 10.1016/j.cstres.2024.10.004. Epub 2024 Oct 18. Cell Stress Chaperones. 2024. PMID: 39426497 Free PMC article.
References
-
- Balchin D., Hayer-Hartl M., Hartl F.U.. In vivo aspects of protein folding and quality control. Science. 2016; 353:aac4354. - PubMed
-
- Kramer G., Shiber A., Bukau B.. Mechanisms of cotranslational maturation of newly synthesized proteins. Annu. Rev. Biochem. 2019; 88:337–364. - PubMed
-
- Zhang Y., Sinning I., Rospert S.. Two chaperones locked in an embrace: structure and function of the ribosome-associated complex RAC. Nat. Struct. Mol. Biol. 2017; 24:611–619. - PubMed
-
- Wiedmann B., Sakai H., Davis T.A., Wiedmann M.. A protein complex required for signal-sequence-specific sorting and translocation. Nature. 1994; 370:434–440. - PubMed
MeSH terms
Substances
Grants and funding
LinkOut - more resources
Full Text Sources