Neuronal tuning to threat exposure remains stable in the mouse prefrontal cortex over multiple days
- PMID: 38206890
- PMCID: PMC10783789
- DOI: 10.1371/journal.pbio.3002475
Neuronal tuning to threat exposure remains stable in the mouse prefrontal cortex over multiple days
Abstract
Intense threat elicits action in the form of active and passive coping. The medial prefrontal cortex (mPFC) executes top-level control over the selection of threat coping strategies, but the dynamics of mPFC activity upon continuing threat encounters remain unexplored. Here, we used 1-photon calcium imaging in mice to probe the activity of prefrontal pyramidal cells during repeated exposure to intense threat in a tail suspension (TS) paradigm. A subset of prefrontal neurons displayed selective activation during TS, which was stably maintained over days. During threat, neurons showed specific tuning to active or passive coping. These responses were unrelated to general motion tuning and persisted over days. Moreover, the neural manifold traversed by low-dimensional population activity remained stable over subsequent days of TS exposure and was preserved across individuals. These data thus reveal a specific, temporally, and interindividually conserved repertoire of prefrontal tuning to behavioral responses under threat.
Copyright: © 2024 Sylte et al. This is an open access article distributed under the terms of the Creative Commons Attribution License, which permits unrestricted use, distribution, and reproduction in any medium, provided the original author and source are credited.
Conflict of interest statement
The authors have declared that no competing interests exist.
Figures
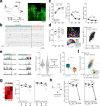
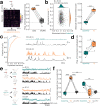
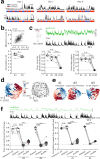
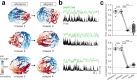
Similar articles
-
Prefrontal-Bed Nucleus Circuit Modulation of a Passive Coping Response Set.J Neurosci. 2019 Feb 20;39(8):1405-1419. doi: 10.1523/JNEUROSCI.1421-18.2018. Epub 2018 Dec 20. J Neurosci. 2019. PMID: 30573644 Free PMC article.
-
Cell-Type-Specific Contributions of Medial Prefrontal Neurons to Flexible Behaviors.J Neurosci. 2018 May 9;38(19):4490-4504. doi: 10.1523/JNEUROSCI.3537-17.2018. Epub 2018 Apr 12. J Neurosci. 2018. PMID: 29650697 Free PMC article.
-
Behavioral State-Dependent Modulation of Prefrontal Cortex Activity by Respiration.J Neurosci. 2023 Jun 28;43(26):4795-4807. doi: 10.1523/JNEUROSCI.2075-22.2023. Epub 2023 Jun 5. J Neurosci. 2023. PMID: 37277176 Free PMC article.
-
Repeated toluene exposure increases the excitability of layer 5 pyramidal neurons in the prefrontal cortex of adolescent rats.Neurotoxicol Teratol. 2018 Jul-Aug;68:27-35. doi: 10.1016/j.ntt.2018.04.006. Epub 2018 Apr 30. Neurotoxicol Teratol. 2018. PMID: 29715503
-
Modulation of azimuth tuning plasticity in rat primary auditory cortex by medial prefrontal cortex.Neuroscience. 2017 Apr 7;347:36-47. doi: 10.1016/j.neuroscience.2017.01.046. Epub 2017 Feb 8. Neuroscience. 2017. PMID: 28188851
References
MeSH terms
Grants and funding
LinkOut - more resources
Full Text Sources