Microphysiological systems for human aging research
- PMID: 38180277
- PMCID: PMC10928588
- DOI: 10.1111/acel.14070
Microphysiological systems for human aging research
Abstract
Recent advances in microphysiological systems (MPS), also known as organs-on-a-chip (OoC), enable the recapitulation of more complex organ and tissue functions on a smaller scale in vitro. MPS therefore provide the potential to better understand human diseases and physiology. To date, numerous MPS platforms have been developed for various tissues and organs, including the heart, liver, kidney, blood vessels, muscle, and adipose tissue. However, only a few studies have explored using MPS platforms to unravel the effects of aging on human physiology and the pathogenesis of age-related diseases. Age is one of the risk factors for many diseases, and enormous interest has been devoted to aging research. As such, a human MPS aging model could provide a more predictive tool to understand the molecular and cellular mechanisms underlying human aging and age-related diseases. These models can also be used to evaluate preclinical drugs for age-related diseases and translate them into clinical settings. Here, we provide a review on the application of MPS in aging research. First, we offer an overview of the molecular, cellular, and physiological changes with age in several tissues or organs. Next, we discuss previous aging models and the current state of MPS for studying human aging and age-related conditions. Lastly, we address the limitations of current MPS and present future directions on the potential of MPS platforms for human aging research.
Keywords: age-related changes; age-related diseases; aging; aging phenotypes; microphysiological systems.
© 2023 The Authors. Aging Cell published by Anatomical Society and John Wiley & Sons Ltd.
Conflict of interest statement
D.‐H.K is a co‐founder and scientific advisory board member at Curi Bio, Inc.
Figures
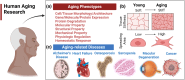
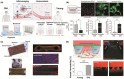
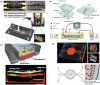
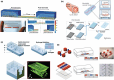
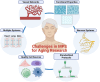
Similar articles
-
Challenges and Future Perspectives in Modeling Neurodegenerative Diseases Using Organ-on-a-Chip Technology.Adv Sci (Weinh). 2024 Aug;11(32):e2403892. doi: 10.1002/advs.202403892. Epub 2024 Jun 23. Adv Sci (Weinh). 2024. PMID: 38922799 Free PMC article. Review.
-
Microphysiological systems to advance human pathophysiology and translational medicine.J Appl Physiol (1985). 2024 Nov 1;137(5):1494-1501. doi: 10.1152/japplphysiol.00087.2024. Epub 2024 Oct 17. J Appl Physiol (1985). 2024. PMID: 39417817 Review.
-
Circadian hormone control in a human-on-a-chip: In vitro biology's ignored component?Exp Biol Med (Maywood). 2017 Nov;242(17):1714-1731. doi: 10.1177/1535370217732766. Exp Biol Med (Maywood). 2017. PMID: 29065796 Free PMC article. Review.
-
Fitting tissue chips and microphysiological systems into the grand scheme of medicine, biology, pharmacology, and toxicology.Exp Biol Med (Maywood). 2017 Oct;242(16):1559-1572. doi: 10.1177/1535370217732765. Exp Biol Med (Maywood). 2017. PMID: 29065799 Free PMC article.
-
Applications of microphysiological systems to disease models in the biopharmaceutical industry: Opportunities and challenges.ALTEX. 2023;40(3):485-518. doi: 10.14573/altex.2204071. Epub 2023 Jan 12. ALTEX. 2023. PMID: 36648096
Cited by
-
Challenges and Future Perspectives in Modeling Neurodegenerative Diseases Using Organ-on-a-Chip Technology.Adv Sci (Weinh). 2024 Aug;11(32):e2403892. doi: 10.1002/advs.202403892. Epub 2024 Jun 23. Adv Sci (Weinh). 2024. PMID: 38922799 Free PMC article. Review.
-
Aging-related changes in the mechanical properties of single cells.Heliyon. 2024 Jun 15;10(12):e32974. doi: 10.1016/j.heliyon.2024.e32974. eCollection 2024 Jun 30. Heliyon. 2024. PMID: 38994100 Free PMC article. Review.
-
Precision Repair of Zone-Specific Meniscal Injuries Using a Tunable Extracellular Matrix-Based Hydrogel System.bioRxiv [Preprint]. 2024 Sep 17:2024.09.12.612723. doi: 10.1101/2024.09.12.612723. bioRxiv. 2024. PMID: 39345590 Free PMC article. Preprint.
References
-
- Achberger, K. , Probst, C. , Haderspeck, J. C. , Bolz, S. , Rogal, J. , Chuchuy, J. , Nikolova, M. , Cora, V. , Antkowiak, L. , Haq, W. , Shen, N. , Schenke‐Layland, K. , Ueffing, M. , Liebau, S. , & Loskill, P. (2019). Merging organoid and organ‐on‐a‐chip technology to generate complex multi‐layer tissue models in a human retina‐on‐a‐chip platform. eLife, 8, e46188. - PMC - PubMed
-
- Aguzzi, A. , & O'Connor, T. (2010). Protein aggregation diseases: Pathogenicity and therapeutic perspectives. Nature Reviews. Drug Discovery, 9, 237–248. - PubMed
Publication types
MeSH terms
Grants and funding
LinkOut - more resources
Full Text Sources
Research Materials