Squishy matters - Corneal mechanobiology in health and disease
- PMID: 38176611
- PMCID: PMC11193890
- DOI: 10.1016/j.preteyeres.2023.101234
Squishy matters - Corneal mechanobiology in health and disease
Abstract
The cornea, as a dynamic and responsive tissue, constantly interacts with mechanical forces in order to maintain its structural integrity, barrier function, transparency and refractive power. Cells within the cornea sense and respond to various mechanical forces that fundamentally regulate their morphology and fate in development, homeostasis and pathophysiology. Corneal cells also dynamically regulate their extracellular matrix (ECM) with ensuing cell-ECM crosstalk as the matrix serves as a dynamic signaling reservoir providing biophysical and biochemical cues to corneal cells. Here we provide an overview of mechanotransduction signaling pathways then delve into the recent advances in corneal mechanobiology, focusing on the interplay between mechanical forces and responses of the corneal epithelial, stromal, and endothelial cells. We also identify species-specific differences in corneal biomechanics and mechanotransduction to facilitate identification of optimal animal models to study corneal wound healing, disease, and novel therapeutic interventions. Finally, we identify key knowledge gaps and therapeutic opportunities in corneal mechanobiology that are pressing for the research community to address especially pertinent within the domains of limbal stem cell deficiency, keratoconus and Fuchs' endothelial corneal dystrophy. By furthering our understanding corneal mechanobiology, we can contextualize discoveries regarding corneal diseases as well as innovative treatments for them.
Keywords: Cell-matrix interactions; Cornea; Corneal wound healing; Mechanobiology; Mechanotransduction; Stiffness.
Copyright © 2023. Published by Elsevier Ltd.
Conflict of interest statement
Declaration of competing interest
Employee: Novartis institutes for biomedical research (NIBR). Work described in the study were from VR’s time in academia and NIBR had no role in study design, execution, planning, funding, interpretation of results, manuscript preparation or decision to publish.
None for ST, BL, MG, and JS.
Figures
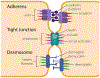
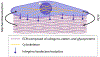
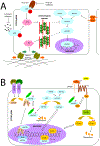
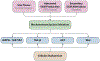
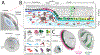
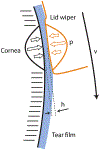
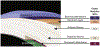
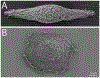
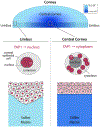
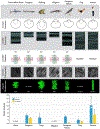
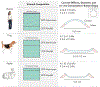
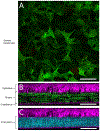
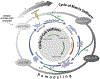
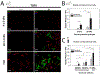
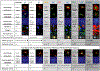
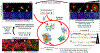
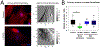
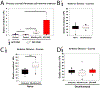
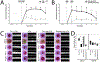
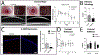
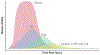
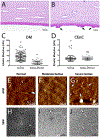
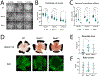
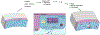
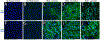
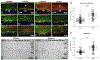
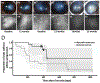
Similar articles
-
Biomechanical relationships between the corneal endothelium and Descemet's membrane.Exp Eye Res. 2016 Nov;152:57-70. doi: 10.1016/j.exer.2016.09.004. Epub 2016 Sep 14. Exp Eye Res. 2016. PMID: 27639516 Free PMC article. Review.
-
Keratoconus and Fuchs' corneal endothelial dystrophy in a patient and her family.Arch Ophthalmol. 1990 Jul;108(7):993-4. doi: 10.1001/archopht.1990.01070090095047. Arch Ophthalmol. 1990. PMID: 2369360
-
Coincident keratoconus and Fuchs' endothelial dystrophy: Dual dystrophies mask progression.Indian J Ophthalmol. 2020 Dec;68(12):3074-3076. doi: 10.4103/ijo.IJO_1783_20. Indian J Ophthalmol. 2020. PMID: 33229712 Free PMC article.
-
Corneal abnormalities early in the course of Fuchs' endothelial dystrophy.Ophthalmology. 2014 Dec;121(12):2325-33. doi: 10.1016/j.ophtha.2014.07.001. Epub 2014 Aug 22. Ophthalmology. 2014. PMID: 25156138 Free PMC article.
-
MicroRNA of Epithelial to Mesenchymal Transition in Fuchs' Endothelial Corneal Dystrophy.Genes (Basel). 2022 Sep 23;13(10):1711. doi: 10.3390/genes13101711. Genes (Basel). 2022. PMID: 36292595 Free PMC article. Review.
Cited by
-
Focus on seed cells: stem cells in 3D bioprinting of corneal grafts.Front Bioeng Biotechnol. 2024 Jul 10;12:1423864. doi: 10.3389/fbioe.2024.1423864. eCollection 2024. Front Bioeng Biotechnol. 2024. PMID: 39050685 Free PMC article. Review.
References
-
- Ablamowicz AF, Nichols JJ, 2016. Ocular surface membrane-associated mucins. Ocul. Surf 14, 331–341. - PubMed
-
- Abrams GA, Bentley E, Nealey PF, Murphy CJ, 2002. Electron microscopy of the canine corneal basement membranes. Cells Tissues Organs 170, 251–257. - PubMed
-
- Abrams GA, Goodman SL, Nealey PF, Franco M, Murphy CJ, 2000a. Nanoscale topography of the basement membrane underlying the corneal epithelium of the rhesus macaque. Cell Tissue Res 299, 39–46. - PubMed
-
- Abrams GA, Schaus SS, Goodman SL, Nealey PF, Murphy CJ, 2000b. Nanoscale topography of the corneal epithelial basement membrane and Descemet’s membrane of the human. Cornea 19, 57–64. - PubMed
-
- Afshari NA, Igo RP Jr., Morris NJ, Stambolian D, Sharma S, Pulagam VL, Dunn S, Stamler JF, Truitt BJ, Rimmler J, Kuot A, Croasdale CR, Qin X, Burdon KP, Riazuddin SA, Mills R, Klebe S, Minear MA, Zhao J, Balajonda E, Rosenwasser GO, Baratz KH, Mootha VV, Patel SV, Gregory SG, Bailey-Wilson JE, Price MO, Price FW Jr., Craig JE, Fingert JH, Gottsch JD, Aldave AJ, Klintworth GK, Lass JH, Li YJ, Iyengar SK, 2017. Genome-wide association study identifies three novel loci in Fuchs endothelial corneal dystrophy. Nat. Commun 8, 14898. - PMC - PubMed