Tumor-on-a-chip models combined with mini-tissues or organoids for engineering tumor tissues
- PMID: 38164155
- PMCID: PMC10750204
- DOI: 10.7150/thno.90093
Tumor-on-a-chip models combined with mini-tissues or organoids for engineering tumor tissues
Abstract
The integration of tumor-on-a-chip technology with mini-tissues or organoids has emerged as a powerful approach in cancer research and drug development. This review provides an extensive examination of the diverse biofabrication methods employed to create mini-tissues, including 3D bioprinting, spheroids, microfluidic systems, and self-assembly techniques using cell-laden hydrogels. Furthermore, it explores various approaches for fabricating organ-on-a-chip platforms. This paper highlights the synergistic potential of combining these technologies to create tumor-on-a-chip models that mimic the complex tumor microenvironment and offer unique insights into cancer biology and therapeutic responses.
Keywords: Biofabrication; Cancer research; Organoids; Tumor microenvironment; Tumor-on-a-chip.
© The author(s).
Conflict of interest statement
Competing Interests: The authors have declared that no competing interest exists.
Figures
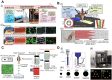
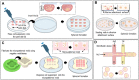
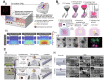
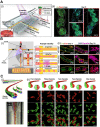
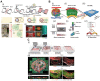
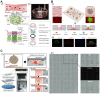
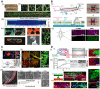
Similar articles
-
3D Biomimetic Models to Reconstitute Tumor Microenvironment In Vitro: Spheroids, Organoids, and Tumor-on-a-Chip.Adv Healthc Mater. 2023 Jul;12(18):e2202609. doi: 10.1002/adhm.202202609. Epub 2023 Mar 29. Adv Healthc Mater. 2023. PMID: 36917657 Free PMC article. Review.
-
Bioprinting of Cells, Organoids and Organs-on-a-Chip Together with Hydrogels Improves Structural and Mechanical Cues.Cells. 2024 Oct 1;13(19):1638. doi: 10.3390/cells13191638. Cells. 2024. PMID: 39404401 Free PMC article. Review.
-
Converging bioprinting and organoids to better recapitulate the tumor microenvironment.Trends Biotechnol. 2024 May;42(5):648-663. doi: 10.1016/j.tibtech.2023.11.006. Epub 2023 Dec 9. Trends Biotechnol. 2024. PMID: 38071145 Review.
-
Strategies for developing complex multi-component in vitro tumor models: Highlights in glioblastoma.Adv Drug Deliv Rev. 2022 Jan;180:114067. doi: 10.1016/j.addr.2021.114067. Epub 2021 Nov 22. Adv Drug Deliv Rev. 2022. PMID: 34822927 Free PMC article. Review.
-
Tissue engineering of the retina: from organoids to microfluidic chips.J Tissue Eng. 2021 Dec 10;12:20417314211059876. doi: 10.1177/20417314211059876. eCollection 2021 Jan-Dec. J Tissue Eng. 2021. PMID: 34917332 Free PMC article. Review.
Cited by
-
Nanomedicine for cancer patient-centered care.MedComm (2020). 2024 Oct 20;5(11):e767. doi: 10.1002/mco2.767. eCollection 2024 Nov. MedComm (2020). 2024. PMID: 39434967 Free PMC article. Review.
-
Tumour organoids and assembloids: Patient-derived cancer avatars for immunotherapy.Clin Transl Med. 2024 Apr;14(4):e1656. doi: 10.1002/ctm2.1656. Clin Transl Med. 2024. PMID: 38664597 Free PMC article. Review.
-
Targeted Cancer Therapy-on-A-Chip.Adv Healthc Mater. 2024 Nov;13(29):e2400833. doi: 10.1002/adhm.202400833. Epub 2024 Aug 5. Adv Healthc Mater. 2024. PMID: 39101627 Free PMC article. Review.
-
Tumor-microenvironment-on-a-chip: the construction and application.Cell Commun Signal. 2024 Oct 23;22(1):515. doi: 10.1186/s12964-024-01884-4. Cell Commun Signal. 2024. PMID: 39438954 Free PMC article. Review.
-
Advancements in Research and Treatment Applications of Patient-Derived Tumor Organoids in Colorectal Cancer.Cancers (Basel). 2024 Jul 26;16(15):2671. doi: 10.3390/cancers16152671. Cancers (Basel). 2024. PMID: 39123399 Free PMC article. Review.
References
-
- Zugazagoitia J, Guedes C, Ponce S, Ferrer I, Molina-Pinelo S, Paz-Ares L. Current challenges in cancer treatment. Clinical therapeutics. 2016;38:1551–66. - PubMed
-
- Imamura Y, Mukohara T, Shimono Y, Funakoshi Y, Chayahara N, Toyoda M. et al. Comparison of 2D-and 3D-culture models as drug-testing platforms in breast cancer. Oncology reports. 2015;33:1837–43. - PubMed
-
- Chen G, Xu Y, Kwok PCL, Kang L. Pharmaceutical applications of 3D printing. Additive Manufacturing. 2020;34:101209.
Publication types
MeSH terms
Substances
LinkOut - more resources
Full Text Sources