Impact of eIF2α phosphorylation on the translational landscape of mouse embryonic stem cells
- PMID: 38159280
- PMCID: PMC10962698
- DOI: 10.1016/j.celrep.2023.113615
Impact of eIF2α phosphorylation on the translational landscape of mouse embryonic stem cells
Abstract
The integrated stress response (ISR) is critical for cell survival under stress. In response to diverse environmental cues, eIF2α becomes phosphorylated, engendering a dramatic change in mRNA translation. The activation of ISR plays a pivotal role in the early embryogenesis, but the eIF2-dependent translational landscape in pluripotent embryonic stem cells (ESCs) is largely unexplored. We employ a multi-omics approach consisting of ribosome profiling, proteomics, and metabolomics in wild-type (eIF2α+/+) and phosphorylation-deficient mutant eIF2α (eIF2αA/A) mouse ESCs (mESCs) to investigate phosphorylated (p)-eIF2α-dependent translational control of naive pluripotency. We show a transient increase in p-eIF2α in the naive epiblast layer of E4.5 embryos. Absence of eIF2α phosphorylation engenders an exit from naive pluripotency following 2i (two chemical inhibitors of MEK1/2 and GSK3α/β) withdrawal. p-eIF2α controls translation of mRNAs encoding proteins that govern pluripotency, chromatin organization, and glutathione synthesis. Thus, p-eIF2α acts as a key regulator of the naive pluripotency gene regulatory network.
Keywords: Stem cell research; embryonic stem cells; p-eIF2α; pluripotency; ribosome profiling; translational control.
Copyright © 2023 The Authors. Published by Elsevier Inc. All rights reserved.
Conflict of interest statement
Declaration of interests The authors declare no competing interests.
Figures
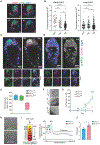
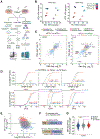
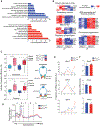
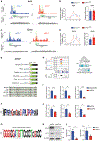
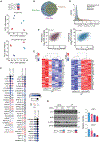
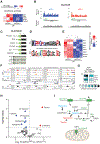
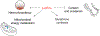
Similar articles
-
Embryonic Stem Cell Growth Factors Regulate eIF2α Phosphorylation.PLoS One. 2015 Sep 25;10(9):e0139076. doi: 10.1371/journal.pone.0139076. eCollection 2015. PLoS One. 2015. PMID: 26406898 Free PMC article.
-
Quantitative subcellular proteomics using SILAC reveals enhanced metabolic buffering in the pluripotent ground state.Stem Cell Res. 2018 Dec;33:135-145. doi: 10.1016/j.scr.2018.09.017. Epub 2018 Sep 24. Stem Cell Res. 2018. PMID: 30352361
-
Translational control during endoplasmic reticulum stress beyond phosphorylation of the translation initiation factor eIF2α.J Biol Chem. 2014 May 2;289(18):12593-611. doi: 10.1074/jbc.M113.543215. Epub 2014 Mar 19. J Biol Chem. 2014. PMID: 24648524 Free PMC article.
-
Endoplasmic reticulum stress and eIF2α phosphorylation: The Achilles heel of pancreatic β cells.Mol Metab. 2017 Jul 12;6(9):1024-1039. doi: 10.1016/j.molmet.2017.06.001. eCollection 2017 Sep. Mol Metab. 2017. PMID: 28951826 Free PMC article. Review.
-
The Integrated Stress Response and Phosphorylated Eukaryotic Initiation Factor 2α in Neurodegeneration.J Neuropathol Exp Neurol. 2020 Feb 1;79(2):123-143. doi: 10.1093/jnen/nlz129. J Neuropathol Exp Neurol. 2020. PMID: 31913484 Free PMC article. Review.
Cited by
-
Metabolic regulation of the hallmarks of stem cell biology.Cell Stem Cell. 2024 Feb 1;31(2):161-180. doi: 10.1016/j.stem.2024.01.003. Cell Stem Cell. 2024. PMID: 38306993 Review.
-
Transfer RNA acetylation regulates in vivo mammalian stress signaling.bioRxiv [Preprint]. 2024 Jul 28:2024.07.25.605208. doi: 10.1101/2024.07.25.605208. bioRxiv. 2024. PMID: 39091849 Free PMC article. Preprint.
-
NPC1 is required for postnatal islet β cell differentiation by maintaining mitochondria turnover.Theranostics. 2024 Feb 24;14(5):2058-2074. doi: 10.7150/thno.90946. eCollection 2024. Theranostics. 2024. PMID: 38505613 Free PMC article.
References
Publication types
MeSH terms
Substances
Grants and funding
LinkOut - more resources
Full Text Sources
Molecular Biology Databases
Miscellaneous