In Vitro Anti-Oxidant, In Vivo Anti-Hyperglycemic, and Untargeted Metabolomics-Aided-In Silico Screening of Macroalgae Lipophilic Extracts for Anti-Diabetes Mellitus and Anti-COVID-19 Potential Metabolites
- PMID: 38132859
- PMCID: PMC10745437
- DOI: 10.3390/metabo13121177
In Vitro Anti-Oxidant, In Vivo Anti-Hyperglycemic, and Untargeted Metabolomics-Aided-In Silico Screening of Macroalgae Lipophilic Extracts for Anti-Diabetes Mellitus and Anti-COVID-19 Potential Metabolites
Abstract
COVID-19 patients with comorbid DM face more severe outcomes, indicating that hyperglycemic conditions exacerbate SARS-CoV-2 infection. Negative side effects from existing hyperglycemia treatments have urged the need for safer compounds. Therefore, sourcing potential compounds from marine resources becomes a new potential approach. Algal lipids are known to possess beneficial activities for human health. However, due to limitations in analyzing large amounts of potential anti-hyperglycemic and anti-COVID-19-related marine metabolites, there is an increasing need for new approaches to reduce risks and costs. Therefore, the main aim of this study was to identify potential compounds in macroalgae Sargassum cristaefolium, Tricleocarpa cylindrica, and Ulva lactuca lipophilic extracts for treating DM and COVID-19 by an integrated approach utilizing in vitro anti-oxidant, in vivo anti-hyperglycemic, and metabolomic-integrated in silico approaches. Among them, S. cristaefolium and T. cylindrica showed potential anti-hyperglycemic activity, with S. cristaefolium showing the highest anti-oxidant activity. A GC-MS-based untargeted metabolomic analysis was used to profile the lipophilic compounds in the extracts followed by an in silico molecular docking analysis to examine the binding affinity of the compounds to anti-DM and anti-COVID-19 targets, e.g., α-amylase, α-glucosidase, ACE2, and TMPRSS2. Notably, this study reveals for the first time that steroid-derived compounds in the macroalgae T. cylindrica had higher binding activity than known ligands for all the targets mentioned. Studies on drug likeliness indicate that these compounds possess favorable drug properties. These findings suggest the potential for these compounds to be further developed to treat COVID-19 patients with comorbid DM. The information in this study would be a basis for further in vitro and in vivo analysis. It would also be useful for the development of these candidate compounds into drug formulations.
Keywords: COVID-19; diabetes mellitus; lipophilic compounds; macroalgae; metabolomics-aided in silico.
Conflict of interest statement
The authors declare no conflict of interest.
Figures
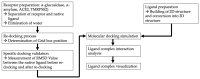
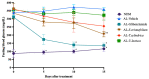
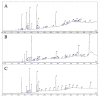
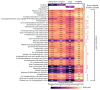
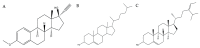
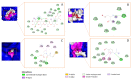
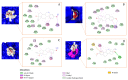
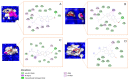
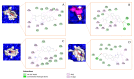
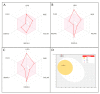
Similar articles
-
Antimicrobial activity of extracts from macroalgae Ulva lactuca against clinically important Staphylococci is impacted by lunar phase of macroalgae harvest.Lett Appl Microbiol. 2016 May;62(5):363-71. doi: 10.1111/lam.12563. Lett Appl Microbiol. 2016. PMID: 26958825
-
Chemical profiling and unraveling of anti-COVID-19 biomarkers of red sage (Lantana camara L.) cultivars using UPLC-MS/MS coupled to chemometric analysis, in vitro study and molecular docking.J Ethnopharmacol. 2022 Jun 12;291:115038. doi: 10.1016/j.jep.2022.115038. Epub 2022 Feb 10. J Ethnopharmacol. 2022. PMID: 35151836 Free PMC article.
-
Translational Metabolomics of Head Injury: Exploring Dysfunctional Cerebral Metabolism with Ex Vivo NMR Spectroscopy-Based Metabolite Quantification.In: Kobeissy FH, editor. Brain Neurotrauma: Molecular, Neuropsychological, and Rehabilitation Aspects. Boca Raton (FL): CRC Press/Taylor & Francis; 2015. Chapter 25. In: Kobeissy FH, editor. Brain Neurotrauma: Molecular, Neuropsychological, and Rehabilitation Aspects. Boca Raton (FL): CRC Press/Taylor & Francis; 2015. Chapter 25. PMID: 26269925 Free Books & Documents. Review.
-
Soybean-associated endophytic fungi as potential source for anti-COVID-19 metabolites supported by docking analysis.J Appl Microbiol. 2021 Sep;131(3):1193-1211. doi: 10.1111/jam.15031. Epub 2021 Mar 9. J Appl Microbiol. 2021. PMID: 33559270 Free PMC article.
-
Covid 19 May Limit the Use of Anti-hyperglycemic Agents. Does it Call for the Development of New Anti-hyperglycemic Agents?Curr Diabetes Rev. 2022;18(3):e060821195364. doi: 10.2174/1573399817666210806114200. Curr Diabetes Rev. 2022. PMID: 34365928 Review.
References
-
- Birman D. Investigation of the Effects of COVID-19 on Different Organs of the Body. Eurasian J. Chem. Med. Pet. Res. 2022;2:24–36.
Grants and funding
LinkOut - more resources
Full Text Sources
Miscellaneous