The dynamics and biophysics of shape formation: Common themes in plant and animal morphogenesis
- PMID: 38113851
- PMCID: PMC10752614
- DOI: 10.1016/j.devcel.2023.11.003
The dynamics and biophysics of shape formation: Common themes in plant and animal morphogenesis
Abstract
The emergence of tissue form in multicellular organisms results from the complex interplay between genetics and physics. In both plants and animals, cells must act in concert to pattern their behaviors. Our understanding of the factors sculpting multicellular form has increased dramatically in the past few decades. From this work, common themes have emerged that connect plant and animal morphogenesis-an exciting connection that solidifies our understanding of the developmental basis of multicellular life. In this review, we will discuss the themes and the underlying principles that connect plant and animal morphogenesis, including the coordination of gene expression, signaling, growth, contraction, and mechanical and geometric feedback.
Copyright © 2023 Elsevier Inc. All rights reserved.
Conflict of interest statement
Declaration of interests The authors declare no competing interests.
Figures
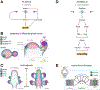
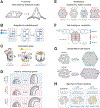
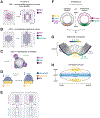
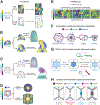
Similar articles
-
Depressing time: Waiting, melancholia, and the psychoanalytic practice of care.In: Kirtsoglou E, Simpson B, editors. The Time of Anthropology: Studies of Contemporary Chronopolitics. Abingdon: Routledge; 2020. Chapter 5. In: Kirtsoglou E, Simpson B, editors. The Time of Anthropology: Studies of Contemporary Chronopolitics. Abingdon: Routledge; 2020. Chapter 5. PMID: 36137063 Free Books & Documents. Review.
-
Australia in 2030: what is our path to health for all?Med J Aust. 2021 May;214 Suppl 8:S5-S40. doi: 10.5694/mja2.51020. Med J Aust. 2021. PMID: 33934362
-
"I've Spent My Whole Life Striving to Be Normal": Internalized Stigma and Perceived Impact of Diagnosis in Autistic Adults.Autism Adulthood. 2023 Dec 1;5(4):423-436. doi: 10.1089/aut.2022.0066. Epub 2023 Dec 12. Autism Adulthood. 2023. PMID: 38116050 Free PMC article.
-
Using Experience Sampling Methodology to Capture Disclosure Opportunities for Autistic Adults.Autism Adulthood. 2023 Dec 1;5(4):389-400. doi: 10.1089/aut.2022.0090. Epub 2023 Dec 12. Autism Adulthood. 2023. PMID: 38116059 Free PMC article.
-
Trends in Surgical and Nonsurgical Aesthetic Procedures: A 14-Year Analysis of the International Society of Aesthetic Plastic Surgery-ISAPS.Aesthetic Plast Surg. 2024 Oct;48(20):4217-4227. doi: 10.1007/s00266-024-04260-2. Epub 2024 Aug 5. Aesthetic Plast Surg. 2024. PMID: 39103642 Review.
Cited by
-
EGFR-dependent actomyosin patterning coordinates morphogenetic movements between tissues.bioRxiv [Preprint]. 2023 Dec 22:2023.12.22.573057. doi: 10.1101/2023.12.22.573057. bioRxiv. 2023. Update in: Dev Cell. 2025 Jan 20;60(2):270-287.e6. doi: 10.1016/j.devcel.2024.10.002. PMID: 38187543 Free PMC article. Updated. Preprint.
-
Drosophila Fog/Cta and T48 pathways have overlapping and distinct contributions to mesoderm invagination.Mol Biol Cell. 2024 May 1;35(5):ar69. doi: 10.1091/mbc.E24-02-0050. Epub 2024 Mar 27. Mol Biol Cell. 2024. PMID: 38536475 Free PMC article.
-
Robust organ size in Arabidopsis is primarily governed by cell growth rather than cell division patterns.Development. 2024 Oct 1;151(19):dev202531. doi: 10.1242/dev.202531. Epub 2024 Oct 10. Development. 2024. PMID: 39324278
-
The Ins and Outs of Homeodomain-Leucine Zipper/Hormone Networks in the Regulation of Plant Development.Int J Mol Sci. 2024 May 23;25(11):5657. doi: 10.3390/ijms25115657. Int J Mol Sci. 2024. PMID: 38891845 Free PMC article. Review.
References
Publication types
MeSH terms
Grants and funding
LinkOut - more resources
Full Text Sources
Miscellaneous