This is a preprint.
Tail Length and E525K Dilated Cardiomyopathy Mutant Alter Human β-Cardiac Myosin Super-Relaxed State
- PMID: 38105932
- PMCID: PMC10723396
- DOI: 10.1101/2023.12.07.570656
Tail Length and E525K Dilated Cardiomyopathy Mutant Alter Human β-Cardiac Myosin Super-Relaxed State
Update in
-
Tail length and E525K dilated cardiomyopathy mutant alter human β-cardiac myosin super-relaxed state.J Gen Physiol. 2024 Jun 3;156(6):e202313522. doi: 10.1085/jgp.202313522. Epub 2024 May 6. J Gen Physiol. 2024. PMID: 38709176 Free PMC article.
Abstract
Dilated cardiomyopathy (DCM) is characterized by impaired cardiac function due to myocardial hypo-contractility and is associated with point mutations in β-cardiac myosin, the molecular motor that powers cardiac contraction. Myocardial function can be modulated through sequestration of myosin motors into an auto-inhibited "super relaxed" state (SRX), which is further stabilized by a structural state known as the "Interacting Heads Motif" (IHM). Therefore, hypo-contractility of DCM myocardium may result from: 1) reduced function of individual myosin, and/or; 2) decreased myosin availability due to increased IHM/SRX stabilization. To define the molecular impact of an established DCM myosin mutation, E525K, we characterized the biochemical and mechanical activity of wild-type (WT) and E525K human β-cardiac myosin constructs that differed in the length of their coiled-coil tail, which dictates their ability to form the IHM/SRX state. Single-headed (S1) and a short-tailed, double-headed (2HEP) myosin constructs exhibited low (~10%) IHM/SRX content, actin-activated ATPase activity of ~5s-1 and fast velocities in unloaded motility assays (~2000nm/s). Double-headed, longer-tailed (15HEP, 25HEP) constructs exhibited higher IHM/SRX content (~90%), and reduced actomyosin ATPase (<1s-1) and velocity (~800nm/s). A simple analytical model suggests that reduced velocities may be attributed to IHM/SRXdependent sequestration of myosin heads. Interestingly, the E525K 15HEP and 25HEP mutants showed no apparent impact on velocity or actomyosin ATPase at low ionic strength. However, at higher ionic strength, the E525K mutation stabilized the IHM/SRX state. Therefore, the E525K-associated DCM human cardiac hypo-contractility may be attributable to reduced myosin head availability caused by enhanced IHM/SRX stability.
Figures
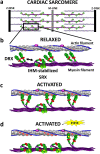
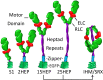
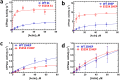
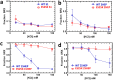
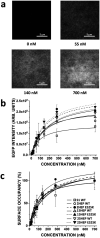
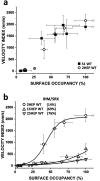
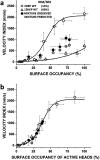
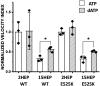
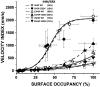
Similar articles
-
Tail length and E525K dilated cardiomyopathy mutant alter human β-cardiac myosin super-relaxed state.J Gen Physiol. 2024 Jun 3;156(6):e202313522. doi: 10.1085/jgp.202313522. Epub 2024 May 6. J Gen Physiol. 2024. PMID: 38709176 Free PMC article.
-
Dilated cardiomyopathy mutation E525K in human beta-cardiac myosin stabilizes the interacting-heads motif and super-relaxed state of myosin.Elife. 2022 Nov 24;11:e77415. doi: 10.7554/eLife.77415. Elife. 2022. PMID: 36422472 Free PMC article.
-
Dilated cardiomyopathy mutation in beta-cardiac myosin enhances actin activation of the power stroke and phosphate release.PNAS Nexus. 2024 Jul 16;3(8):pgae279. doi: 10.1093/pnasnexus/pgae279. eCollection 2024 Aug. PNAS Nexus. 2024. PMID: 39108304 Free PMC article.
-
Cardiac myosin super relaxation (SRX): a perspective on fundamental biology, human disease and therapeutics.Biol Open. 2021 Feb 15;10(2):bio057646. doi: 10.1242/bio.057646. Biol Open. 2021. PMID: 33589442 Free PMC article. Review.
-
Hypertrophic cardiomyopathy and the myosin mesa: viewing an old disease in a new light.Biophys Rev. 2018 Feb;10(1):27-48. doi: 10.1007/s12551-017-0274-6. Epub 2017 Jul 17. Biophys Rev. 2018. PMID: 28717924 Free PMC article. Review.
References
Publication types
Grants and funding
LinkOut - more resources
Full Text Sources