Unexpected Differences in the Speed of Non-Malignant versus Malignant Cell Migration Reveal Differential Basal Intracellular ATP Levels
- PMID: 38067222
- PMCID: PMC10705159
- DOI: 10.3390/cancers15235519
Unexpected Differences in the Speed of Non-Malignant versus Malignant Cell Migration Reveal Differential Basal Intracellular ATP Levels
Abstract
Cellular locomotion is required for survival, fertility, proper embryonic development, regeneration, and wound healing. Cell migration is a major component of metastasis, which accounts for two-thirds of all solid tumor deaths. While many studies have demonstrated increased energy requirements, metabolic rates, and migration of cancer cells compared with normal cells, few have systematically compared normal and cancer cell migration as well as energy requirements side by side. Thus, we investigated how non-malignant and malignant cells migrate, utilizing several cell lines from the breast and lung. Initial screening was performed in an unbiased high-throughput manner for the ability to migrate/invade on collagen and/or Matrigel. We unexpectedly observed that all the non-malignant lung cells moved significantly faster than cells derived from lung tumors regardless of the growth media used. Given the paradigm-shifting nature of our discovery, we pursued the mechanisms that could be responsible. Neither mass, cell doubling, nor volume accounted for the individual speed and track length of the normal cells. Non-malignant cells had higher levels of intracellular ATP at premigratory-wound induction stages. Meanwhile, cancer cells also increased intracellular ATP at premigratory-wound induction, but not to the levels of the normal cells, indicating the possibility for further therapeutic investigation.
Keywords: cancer cell migration; cell migration; normal epithelial cell motility.
Conflict of interest statement
The authors declare no conflict of interest. The funders had no role in the design of the study; in the collection, analyses, or interpretation of data; in the writing of the manuscript; or in the decision to publish the results.
Figures
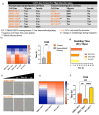
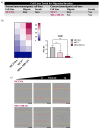
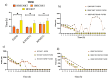
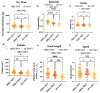
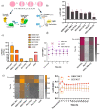
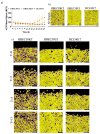
Similar articles
-
The matrix environmental and cell mechanical properties regulate cell migration and contribute to the invasive phenotype of cancer cells.Rep Prog Phys. 2019 Jun;82(6):064602. doi: 10.1088/1361-6633/ab1628. Epub 2019 Apr 4. Rep Prog Phys. 2019. PMID: 30947151 Review.
-
[Identification of a new pro-invasion factor in tumor microenvironment: progress in function and mechanism of extracellular ATP].Beijing Da Xue Xue Bao Yi Xue Ban. 2017 Apr 18;49(2):188-195. Beijing Da Xue Xue Bao Yi Xue Ban. 2017. PMID: 28416823 Chinese.
-
Ovalitenone Inhibits the Migration of Lung Cancer Cells via the Suppression of AKT/mTOR and Epithelial-to-Mesenchymal Transition.Molecules. 2021 Jan 26;26(3):638. doi: 10.3390/molecules26030638. Molecules. 2021. PMID: 33530617 Free PMC article.
-
Integrins and ion channels in cell migration: implications for neuronal development, wound healing and metastatic spread.Adv Exp Med Biol. 2010;674:107-23. doi: 10.1007/978-1-4419-6066-5_10. Adv Exp Med Biol. 2010. PMID: 20549944 Review.
-
Tumor cell locomotion: differential dynamics of spontaneous and induced migration in a 3D collagen matrix.Exp Cell Res. 2004 Aug 1;298(1):178-87. doi: 10.1016/j.yexcr.2004.04.001. Exp Cell Res. 2004. PMID: 15242772
References
-
- LeBleu V.S., O’Connell J.T., Herrera K.N.G., Wikman H., Pantel K., Haigis M.C., de Carvalho F.M., Damascena A., Chinen L.T.D., Rocha R.M., et al. PGC-1alpha mediates mitochondrial biogenesis and oxidative phosphorylation in cancer cells to promote metastasis. Nat. Cell Biol. 2014;16:992–1003. doi: 10.1038/ncb3039. - DOI - PMC - PubMed
-
- Pacheco-Velazquez S.C., Robledo-Cadena D.X., Hernandez-Resendiz I., Gallardo-Perez J.C., Moreno-Sanchez R., Rodriguez-Enriquez S. Energy Metabolism Drugs Block Triple Negative Breast Metastatic Cancer Cell Phenotype. Mol. Pharm. 2018;15:2151–2164. doi: 10.1021/acs.molpharmaceut.8b00015. - DOI - PubMed
Grants and funding
LinkOut - more resources
Full Text Sources