Structural basis of substrate progression through the bacterial chaperonin cycle
- PMID: 38064510
- PMCID: PMC10723157
- DOI: 10.1073/pnas.2308933120
Structural basis of substrate progression through the bacterial chaperonin cycle
Abstract
The bacterial chaperonin GroEL-GroES promotes protein folding through ATP-regulated cycles of substrate protein binding, encapsulation, and release. Here, we have used cryoEM to determine structures of GroEL, GroEL-ADP·BeF3, and GroEL-ADP·AlF3-GroES all complexed with the model substrate Rubisco. Our structures provide a series of snapshots that show how the conformation and interactions of non-native Rubisco change as it proceeds through the GroEL-GroES reaction cycle. We observe specific charged and hydrophobic GroEL residues forming strong initial contacts with non-native Rubisco. Binding of ATP or ADP·BeF3 to GroEL-Rubisco results in the formation of an intermediate GroEL complex displaying striking asymmetry in the ATP/ADP·BeF3-bound ring. In this ring, four GroEL subunits bind Rubisco and the other three are in the GroES-accepting conformation, suggesting how GroEL can recruit GroES without releasing bound substrate. Our cryoEM structures of stalled GroEL-ADP·AlF3-Rubisco-GroES complexes show Rubisco folding intermediates interacting with GroEL-GroES via different sets of residues.
Keywords: CryoEM; Rubisco; chaperonins; protein folding.
Conflict of interest statement
Competing interests statement:M.C.D. was an employee of SPT Labtech, the company that manufactures Chameleon systems.
Figures
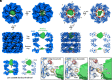
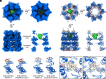
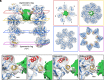
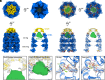
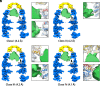
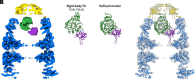
Similar articles
-
GroEL/GroES cycling: ATP binds to an open ring before substrate protein favoring protein binding and production of the native state.Proc Natl Acad Sci U S A. 2009 Dec 1;106(48):20264-9. doi: 10.1073/pnas.0911556106. Epub 2009 Nov 13. Proc Natl Acad Sci U S A. 2009. PMID: 19915138 Free PMC article.
-
Triggering protein folding within the GroEL-GroES complex.J Biol Chem. 2008 Nov 14;283(46):32003-13. doi: 10.1074/jbc.M802898200. Epub 2008 Sep 9. J Biol Chem. 2008. PMID: 18782766 Free PMC article.
-
No evidence for a forced-unfolding mechanism during ATP/GroES binding to substrate-bound GroEL: no observable protection of metastable Rubisco intermediate or GroEL-bound Rubisco from tritium exchange.FEBS Lett. 2005 Feb 14;579(5):1183-6. doi: 10.1016/j.febslet.2005.01.013. FEBS Lett. 2005. PMID: 15710410
-
GroEL and the GroEL-GroES Complex.Subcell Biochem. 2017;83:483-504. doi: 10.1007/978-3-319-46503-6_17. Subcell Biochem. 2017. PMID: 28271487 Review.
-
Reaction Cycle of Chaperonin GroEL via Symmetric "Football" Intermediate.J Mol Biol. 2015 Sep 11;427(18):2912-8. doi: 10.1016/j.jmb.2015.04.007. Epub 2015 Apr 18. J Mol Biol. 2015. PMID: 25900372 Review.
Cited by
-
The role of molecular chaperone CCT/TRiC in translation elongation: A literature review.Heliyon. 2024 Apr 1;10(7):e29029. doi: 10.1016/j.heliyon.2024.e29029. eCollection 2024 Apr 15. Heliyon. 2024. PMID: 38596045 Free PMC article. Review.
-
Asymmetric apical domain states of mitochondrial Hsp60 coordinate substrate engagement and chaperonin assembly.Nat Struct Mol Biol. 2024 Dec;31(12):1848-1858. doi: 10.1038/s41594-024-01352-0. Epub 2024 Jul 1. Nat Struct Mol Biol. 2024. PMID: 38951622 Free PMC article.
-
Visualizing chaperonin function in situ by cryo-electron tomography.Nature. 2024 Sep;633(8029):459-464. doi: 10.1038/s41586-024-07843-w. Epub 2024 Aug 21. Nature. 2024. PMID: 39169181 Free PMC article.
References
-
- Horwich A. L., Fenton W. A., Chaperonin-assisted protein folding: A chronologue. Q. Rev. Biophys. 53, e4 (2020). - PubMed
-
- Braig K., et al. , The crystal structure of the bacterial chaperonln GroEL at 2.8 Å. Nature 371, 578–586 (1994). - PubMed
-
- Fenton W. A., Kashi Y., Furtak K., Norwich A. L., Residues in chaperonin GroEL required for polypeptide binding and release. Nature 371, 614–619 (1994). - PubMed
-
- Hunt J. F., Weaver A. J., Landry S. J., Gierasch L., Deisenhofer J., The crystal structure of the GroES co-chaperonin at 2.8 Å resolution. Nature 379, 37–45 (1996). - PubMed
MeSH terms
Substances
Grants and funding
LinkOut - more resources
Full Text Sources
Research Materials