Direct evidence of lipid transport by the Drs2-Cdc50 flippase upon truncation of its terminal regions
- PMID: 38063271
- PMCID: PMC10895448
- DOI: 10.1002/pro.4855
Direct evidence of lipid transport by the Drs2-Cdc50 flippase upon truncation of its terminal regions
Abstract
P4-ATPases in complex with Cdc50 subunits are lipid flippases that couple ATP hydrolysis with lipid transport to the cytoplasmic leaflet of membranes to create lipid asymmetry. Such vectorial transport has been shown to contribute to vesicle formation in the late secretory pathway. Some flippases are regulated by autoinhibitory regions that can be destabilized by protein kinase-mediated phosphorylation and possibly by binding of cytosolic proteins. In addition, the binding of lipids to flippases may also induce conformational changes required for the activity of these transporters. Here, we address the role of phosphatidylinositol-4-phosphate (PI4P) and the terminal autoinhibitory tails on the lipid flipping activity of the yeast lipid flippase Drs2-Cdc50. By functionally reconstituting the full-length and truncated forms of Drs2 in a 1:1 complex with the Cdc50 subunit, we provide compelling evidence that lipid flippase activity is exclusively detected for the truncated Drs2 variant and is dependent on the presence of the phosphoinositide PI4P. These findings highlight the critical role of phosphoinositides as lipid co-factors in the regulation of lipid transport by the Drs2-Cdc50 flippase.
Keywords: P4-ATPase; lipid flippase; membrane transporter; phosphoinositides; reconstitution.
© 2023 The Authors. Protein Science published by Wiley Periodicals LLC on behalf of The Protein Society.
Conflict of interest statement
The authors declare that they have no conflicts of interest with regard to the contents of this article.
Figures
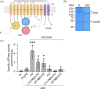
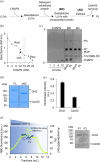
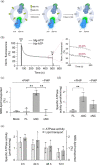
Similar articles
-
High phosphatidylinositol 4-phosphate (PI4P)-dependent ATPase activity for the Drs2p-Cdc50p flippase after removal of its N- and C-terminal extensions.J Biol Chem. 2017 May 12;292(19):7954-7970. doi: 10.1074/jbc.M116.751487. Epub 2017 Mar 16. J Biol Chem. 2017. PMID: 28302728 Free PMC article.
-
A high-yield co-expression system for the purification of an intact Drs2p-Cdc50p lipid flippase complex, critically dependent on and stabilized by phosphatidylinositol-4-phosphate.PLoS One. 2014 Nov 13;9(11):e112176. doi: 10.1371/journal.pone.0112176. eCollection 2014. PLoS One. 2014. PMID: 25393116 Free PMC article.
-
Auto-inhibition of Drs2p, a yeast phospholipid flippase, by its carboxyl-terminal tail.J Biol Chem. 2013 Nov 1;288(44):31807-15. doi: 10.1074/jbc.M113.481986. Epub 2013 Sep 17. J Biol Chem. 2013. PMID: 24045945 Free PMC article.
-
P4-ATPases as Phospholipid Flippases-Structure, Function, and Enigmas.Front Physiol. 2016 Jul 8;7:275. doi: 10.3389/fphys.2016.00275. eCollection 2016. Front Physiol. 2016. PMID: 27458383 Free PMC article. Review.
-
Consensus, controversies, and conundrums of P4-ATPases: The emerging face of eukaryotic lipid flippases.J Biol Chem. 2024 Jun;300(6):107387. doi: 10.1016/j.jbc.2024.107387. Epub 2024 May 17. J Biol Chem. 2024. PMID: 38763336 Free PMC article. Review.
References
-
- Azouaoui H, Montigny C, Ash MR, Fijalkowski F, Jacquot A, Grønberg C et al. A high‐yield co‐expression system for the purification of an intact Drs2p‐Cdc50p lipid flippase complex, critically dependent on and stabilized by phosphatidylinositol‐4‐phosphate. PLoS One. 2014;9(11):e112176. - PMC - PubMed
-
- Azouaoui H, Montigny C, Dieudonné T, Champeil P, Jacquot A, Vázquez‐Ibar JL, et al. High phosphatidylinositol 4‐phosphate (PI4P)‐dependent ATPase activity for the Drs2p‐Cdc50p flippase after removal of its N‐ and C‐terminal extensions. J Biol Chem. 2017;292(19):7954–7970. 10.1074/jbc.M116.751487 - DOI - PMC - PubMed
Grants and funding
LinkOut - more resources
Full Text Sources