Mechanisms and functions of intestinal vascular specialization
- PMID: 38051275
- PMCID: PMC10697212
- DOI: 10.1084/jem.20222008
Mechanisms and functions of intestinal vascular specialization
Abstract
The intestinal vasculature has been studied for the last 100 years, and its essential role in absorbing and distributing ingested nutrients is well known. Recently, fascinating new insights into the organization, molecular mechanisms, and functions of intestinal vessels have emerged. These include maintenance of intestinal epithelial cell function, coping with microbiota-induced inflammatory pressure, recruiting gut-specific immune cells, and crosstalk with other organs. Intestinal function is also regulated at the systemic and cellular levels, such that the postprandial hyperemic response can direct up to 30% of systemic blood to gut vessels, while micron-sized endothelial cell fenestrations are necessary for nutrient uptake. In this review, we will highlight past discoveries made about intestinal vasculature in the context of new findings of molecular mechanisms underpinning gut function. Such comprehensive understanding of the system will pave the way to breakthroughs in nutrient uptake optimization, drug delivery efficiency, and treatment of human diseases.
© 2023 Bernier-Latmani et al.
Conflict of interest statement
Disclosures: The authors declare no competing interests exist.
Figures

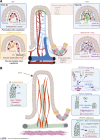
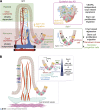
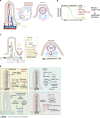
Similar articles
-
Dietary nutrients shape gut microbes and intestinal mucosa via epigenetic modifications.Crit Rev Food Sci Nutr. 2022;62(3):783-797. doi: 10.1080/10408398.2020.1828813. Epub 2020 Oct 12. Crit Rev Food Sci Nutr. 2022. PMID: 33043708
-
Altered intestinal epithelial nutrient transport: an underappreciated factor in obesity modulated by diet and microbiota.Biochem J. 2021 Mar 12;478(5):975-995. doi: 10.1042/BCJ20200902. Biochem J. 2021. PMID: 33661278 Review.
-
Nutrients Mediate Intestinal Bacteria-Mucosal Immune Crosstalk.Front Immunol. 2018 Jan 24;9:5. doi: 10.3389/fimmu.2018.00005. eCollection 2018. Front Immunol. 2018. PMID: 29416535 Free PMC article. Review.
-
ADAMTS18+ villus tip telocytes maintain a polarized VEGFA signaling domain and fenestrations in nutrient-absorbing intestinal blood vessels.Nat Commun. 2022 Jul 9;13(1):3983. doi: 10.1038/s41467-022-31571-2. Nat Commun. 2022. PMID: 35810168 Free PMC article.
-
Intestinal epithelial cell-specific IGF1 promotes the expansion of intestinal stem cells during epithelial regeneration and functions on the intestinal immune homeostasis.Am J Physiol Endocrinol Metab. 2018 Oct 1;315(4):E638-E649. doi: 10.1152/ajpendo.00022.2018. Epub 2018 May 21. Am J Physiol Endocrinol Metab. 2018. PMID: 29783855
Cited by
-
Distal Immunization and Systemic Cytokines Establish a Transient Immune Alert State in the Intestine.J Immunol. 2024 Aug 1;213(3):373-383. doi: 10.4049/jimmunol.2400209. J Immunol. 2024. PMID: 38884660
-
Transport functions of intestinal lymphatic vessels.Nat Rev Gastroenterol Hepatol. 2025 Feb;22(2):127-145. doi: 10.1038/s41575-024-00996-z. Epub 2024 Nov 4. Nat Rev Gastroenterol Hepatol. 2025. PMID: 39496888 Review.
-
Compartmentalization of the inflammatory response during bacterial sepsis and severe COVID-19.J Intensive Med. 2024 Feb 27;4(3):326-340. doi: 10.1016/j.jointm.2024.01.001. eCollection 2024 Jul. J Intensive Med. 2024. PMID: 39035623 Free PMC article. Review.
-
Lymphatic System Development and Function.Curr Cardiol Rep. 2024 Nov;26(11):1209-1219. doi: 10.1007/s11886-024-02120-8. Epub 2024 Aug 22. Curr Cardiol Rep. 2024. PMID: 39172295 Review.
References
Publication types
MeSH terms
Grants and funding
LinkOut - more resources
Full Text Sources
Miscellaneous