Genetic risk converges on regulatory networks mediating early type 2 diabetes
- PMID: 38049589
- PMCID: PMC11374460
- DOI: 10.1038/s41586-023-06693-2
Genetic risk converges on regulatory networks mediating early type 2 diabetes
Abstract
Type 2 diabetes mellitus (T2D), a major cause of worldwide morbidity and mortality, is characterized by dysfunction of insulin-producing pancreatic islet β cells1,2. T2D genome-wide association studies (GWAS) have identified hundreds of signals in non-coding and β cell regulatory genomic regions, but deciphering their biological mechanisms remains challenging3-5. Here, to identify early disease-driving events, we performed traditional and multiplexed pancreatic tissue imaging, sorted-islet cell transcriptomics and islet functional analysis of early-stage T2D and control donors. By integrating diverse modalities, we show that early-stage T2D is characterized by β cell-intrinsic defects that can be proportioned into gene regulatory modules with enrichment in signals of genetic risk. After identifying the β cell hub gene and transcription factor RFX6 within one such module, we demonstrated multiple layers of genetic risk that converge on an RFX6-mediated network to reduce insulin secretion by β cells. RFX6 perturbation in primary human islet cells alters β cell chromatin architecture at regions enriched for T2D GWAS signals, and population-scale genetic analyses causally link genetically predicted reduced RFX6 expression with increased T2D risk. Understanding the molecular mechanisms of complex, systemic diseases necessitates integration of signals from multiple molecules, cells, organs and individuals, and thus we anticipate that this approach will be a useful template to identify and validate key regulatory networks and master hub genes for other diseases or traits using GWAS data.
© 2023. The Author(s), under exclusive licence to Springer Nature Limited.
Conflict of interest statement
Figures
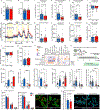
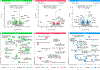
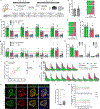
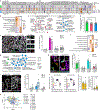
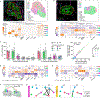
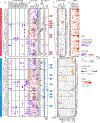
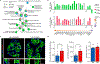
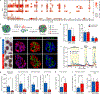
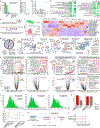
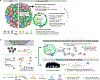
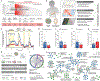
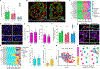
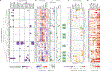
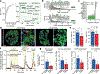
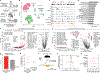
Similar articles
-
Single-cell ATAC-Seq in human pancreatic islets and deep learning upscaling of rare cells reveals cell-specific type 2 diabetes regulatory signatures.Mol Metab. 2020 Feb;32:109-121. doi: 10.1016/j.molmet.2019.12.006. Epub 2019 Dec 20. Mol Metab. 2020. PMID: 32029221 Free PMC article.
-
Genetic regulatory signatures underlying islet gene expression and type 2 diabetes.Proc Natl Acad Sci U S A. 2017 Feb 28;114(9):2301-2306. doi: 10.1073/pnas.1621192114. Epub 2017 Feb 13. Proc Natl Acad Sci U S A. 2017. PMID: 28193859 Free PMC article.
-
Human pancreatic islet three-dimensional chromatin architecture provides insights into the genetics of type 2 diabetes.Nat Genet. 2019 Jul;51(7):1137-1148. doi: 10.1038/s41588-019-0457-0. Epub 2019 Jun 28. Nat Genet. 2019. PMID: 31253982 Free PMC article.
-
(Epi)genomic heterogeneity of pancreatic islet function and failure in type 2 diabetes.Mol Metab. 2019 Sep;27S(Suppl):S15-S24. doi: 10.1016/j.molmet.2019.06.002. Mol Metab. 2019. PMID: 31500827 Free PMC article. Review.
-
Understanding Genetic Heterogeneity in Type 2 Diabetes by Delineating Physiological Phenotypes: SIRT1 and its Gene Network in Impaired Insulin Secretion.Rev Diabet Stud. 2016 Spring;13(1):17-34. doi: 10.1900/RDS.2016.13.17. Epub 2016 May 10. Rev Diabet Stud. 2016. PMID: 27563694 Free PMC article. Review.
Cited by
-
Structure, interaction and nervous connectivity of beta cell primary cilia.Nat Commun. 2024 Oct 24;15(1):9168. doi: 10.1038/s41467-024-53348-5. Nat Commun. 2024. PMID: 39448638 Free PMC article.
-
CNTools: A computational toolbox for cellular neighborhood analysis from multiplexed images.PLoS Comput Biol. 2024 Aug 28;20(8):e1012344. doi: 10.1371/journal.pcbi.1012344. eCollection 2024 Aug. PLoS Comput Biol. 2024. PMID: 39196899 Free PMC article.
-
Thermal Denaturation of Fresh Frozen Tissue Enhances Mass Spectrometry Detection of Peptides.Anal Chem. 2024 Oct 22;96(42):16861-16870. doi: 10.1021/acs.analchem.4c03625. Epub 2024 Oct 11. Anal Chem. 2024. PMID: 39392310 Free PMC article.
-
RFX6 haploinsufficiency predisposes to diabetes through impaired beta cell function.Diabetologia. 2024 Aug;67(8):1642-1662. doi: 10.1007/s00125-024-06163-y. Epub 2024 May 14. Diabetologia. 2024. PMID: 38743124 Free PMC article.
-
Initial clinical manifestations in a young male with RFX6-variant-associated diabetes.Clin Pediatr Endocrinol. 2024 Oct;33(4):224-228. doi: 10.1297/cpe.2024-0016. Epub 2024 Jul 20. Clin Pediatr Endocrinol. 2024. PMID: 39359667 Free PMC article.
References
-
- Kahn SE, Hull RL & Utzschneider KM Mechanisms linking obesity to insulin resistance and type 2 diabetes. Nature 444, 840–846 (2006). - PubMed
MeSH terms
Substances
Grants and funding
- UC4 DK104218/DK/NIDDK NIH HHS/United States
- UC4 DK104211/DK/NIDDK NIH HHS/United States
- P30 DK058404/DK/NIDDK NIH HHS/United States
- U01 DK120456/DK/NIDDK NIH HHS/United States
- UC4 DK112232/DK/NIDDK NIH HHS/United States
- R01 DK129469/DK/NIDDK NIH HHS/United States
- P30 CA068485/CA/NCI NIH HHS/United States
- UC4 DK112217/DK/NIDDK NIH HHS/United States
- T32 GM007544/GM/NIGMS NIH HHS/United States
- R24 DK106755/DK/NIDDK NIH HHS/United States
- R01 DK117147/DK/NIDDK NIH HHS/United States
- U01 DK123716/DK/NIDDK NIH HHS/United States
- U01 DK135017/DK/NIDDK NIH HHS/United States
- F30 DK134041/DK/NIDDK NIH HHS/United States
- R01 HL163262/HL/NHLBI NIH HHS/United States
- U01 DK112217/DK/NIDDK NIH HHS/United States
- R01 HL142302/HL/NHLBI NIH HHS/United States
- UC4 DK098085/DK/NIDDK NIH HHS/United States
- UC4 DK108120/DK/NIDDK NIH HHS/United States
- U24 DK098085/DK/NIDDK NIH HHS/United States
- R01 DK127084/DK/NIDDK NIH HHS/United States
- T32 GM007347/GM/NIGMS NIH HHS/United States
- I01 BX000666/BX/BLRD VA/United States
- P30 DK020593/DK/NIDDK NIH HHS/United States
- R01 DK117960/DK/NIDDK NIH HHS/United States
- U01 DK123743/DK/NIDDK NIH HHS/United States
- F30 DK118830/DK/NIDDK NIH HHS/United States
- UM1 DK126185/DK/NIDDK NIH HHS/United States
- IK2 BX005910/BX/BLRD VA/United States
- U01 DK104218/DK/NIDDK NIH HHS/United States
- U54 EY032442/EY/NEI NIH HHS/United States
LinkOut - more resources
Full Text Sources
Medical