Preclinical long-term safety of intraspinal transplantation of human dorsal spinal GABA neural progenitor cells
- PMID: 38026209
- PMCID: PMC10661464
- DOI: 10.1016/j.isci.2023.108306
Preclinical long-term safety of intraspinal transplantation of human dorsal spinal GABA neural progenitor cells
Abstract
Human pluripotent stem cell (hPSC)-derived neurons have shown promise in treating spinal cord injury (SCI). We previously showed that hPSC-derived dorsal spinal γ-aminobutyric acid (GABA) neurons can alleviate spasticity and promote locomotion in rats with SCI, but their long-term safety remains elusive. Here, we characterized the long-term fate and safety of human dorsal spinal GABA neural progenitor cells (NPCs) in naive rats over one year. All grafted NPCs had undergone differentiation, yielding mainly neurons and astrocytes. Fully mature human neurons grew many axons and formed numerous synapses with rat neural circuits, together with mature human astrocytes that structurally integrated into the rat spinal cord. The sensorimotor function of rats was not impaired by intraspinal transplantation, even when human neurons were activated or inhibited by designer receptors exclusively activated by designer drugs (DREADDs). These findings represent a significant step toward the clinical translation of human spinal neuron transplantation for treating SCI.
Keywords: Biological sciences; Neuroscience.
© 2023 The Author(s).
Conflict of interest statement
The authors declare no competing interests.
Figures
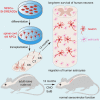
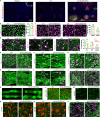
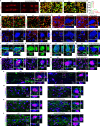
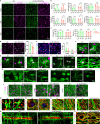
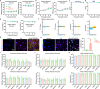
Similar articles
-
Human spinal GABA neurons survive and mature in the injured nonhuman primate spinal cord.Stem Cell Reports. 2023 Feb 14;18(2):439-448. doi: 10.1016/j.stemcr.2022.12.016. Epub 2023 Jan 19. Stem Cell Reports. 2023. PMID: 36669493 Free PMC article.
-
Human spinal GABA neurons alleviate spasticity and improve locomotion in rats with spinal cord injury.Cell Rep. 2021 Mar 23;34(12):108889. doi: 10.1016/j.celrep.2021.108889. Cell Rep. 2021. PMID: 33761348
-
A review of regenerative therapy for spinal cord injury using human iPS cells.N Am Spine Soc J. 2022 Nov 19;13:100184. doi: 10.1016/j.xnsj.2022.100184. eCollection 2023 Mar. N Am Spine Soc J. 2022. PMID: 36479183 Free PMC article.
-
Human stem cell-derived neurons and neural circuitry therapeutics: Next frontier in spinal cord injury repair.Exp Biol Med (Maywood). 2022 Dec;247(23):2142-2151. doi: 10.1177/15353702221114099. Epub 2022 Aug 16. Exp Biol Med (Maywood). 2022. PMID: 35974701 Free PMC article. Review.
-
Regenerative replacement of neural cells for treatment of spinal cord injury.Expert Opin Biol Ther. 2021 Nov;21(11):1411-1427. doi: 10.1080/14712598.2021.1914582. Epub 2021 Apr 26. Expert Opin Biol Ther. 2021. PMID: 33830863 Review.
References
-
- Ribeiro B.F., da Cruz B.C., de Sousa B.M., Correia P.D., David N., Rocha C., Almeida R.D., Ribeiro da Cunha M., Marques Baptista A.A., Vieira S.I. Cell therapies for spinal cord injury: a review of the clinical trials and cell-type therapeutic potential. Brain. 2023;146:2672–2693. doi: 10.1093/brain/awad047. - DOI - PubMed
-
- Huang H., Chen L., Moviglia G., Sharma A., Al Zoubi Z.M., He X., Chen D. Advances and prospects of cell therapy for spinal cord injury patients. J. Neurorestoratol. 2022;10:13–30. doi: 10.26599/Jnr.2022.9040007. - DOI
LinkOut - more resources
Full Text Sources