Role and therapeutic potential of DEAD-box RNA helicase family in colorectal cancer
- PMID: 38023215
- PMCID: PMC10654640
- DOI: 10.3389/fonc.2023.1278282
Role and therapeutic potential of DEAD-box RNA helicase family in colorectal cancer
Abstract
Colorectal cancer (CRC) is the third most commonly diagnosed and the second cancer-related death worldwide, leading to more than 0.9 million deaths every year. Unfortunately, this disease is changing rapidly to a younger age, and in a more advanced stage when diagnosed. The DEAD-box RNA helicase proteins are the largest family of RNA helicases so far. They regulate almost every aspect of RNA physiological processes, including RNA transcription, editing, splicing and transport. Aberrant expression and critical roles of the DEAD-box RNA helicase proteins have been found in CRC. In this review, we first summarize the protein structure, cellular distribution, and diverse biological functions of DEAD-box RNA helicases. Then, we discuss the distinct roles of DEAD-box RNA helicase family in CRC and describe the cellular mechanism of actions based on recent studies, with an aim to provide future strategies for the treatment of CRC.
Keywords: DEAD-box RNA helicases; cellular distribution; colorectal cancer; mechanism; physiological role.
Copyright © 2023 Zheng, Chen, Ling, Cheng and Ye.
Conflict of interest statement
The authors declare that the research was conducted in the absence of any commercial or financial relationships that could be construed as a potential conflict of interest.
Figures

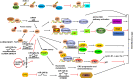
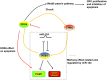
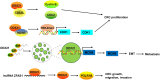
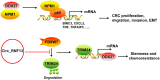
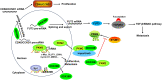
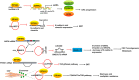
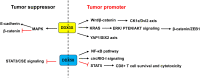
Similar articles
-
The DEAD-box protein family of RNA helicases: sentinels for a myriad of cellular functions with emerging roles in tumorigenesis.Int J Clin Oncol. 2021 May;26(5):795-825. doi: 10.1007/s10147-021-01892-1. Epub 2021 Mar 3. Int J Clin Oncol. 2021. PMID: 33656655 Review.
-
Genera specific distribution of DEAD-box RNA helicases in cyanobacteria.Microb Genom. 2021 Mar;7(3):mgen000517. doi: 10.1099/mgen.0.000517. Epub 2021 Feb 4. Microb Genom. 2021. PMID: 33539277 Free PMC article.
-
DEAD-Box Helicases: Sensors, Regulators, and Effectors for Antiviral Defense.Viruses. 2020 Feb 5;12(2):181. doi: 10.3390/v12020181. Viruses. 2020. PMID: 32033386 Free PMC article. Review.
-
Synthetic lethal interactions of DEAD/H-box helicases as targets for cancer therapy.Front Oncol. 2023 Jan 26;12:1087989. doi: 10.3389/fonc.2022.1087989. eCollection 2022. Front Oncol. 2023. PMID: 36761420 Free PMC article. Review.
-
Oncogenic splicing abnormalities induced by DEAD-Box Helicase 56 amplification in colorectal cancer.Cancer Sci. 2019 Oct;110(10):3132-3144. doi: 10.1111/cas.14163. Epub 2019 Aug 29. Cancer Sci. 2019. PMID: 31390121 Free PMC article.
References
Publication types
Grants and funding
LinkOut - more resources
Full Text Sources