SARS-CoV-2 and Epstein-Barr Virus-like Particles Associate and Fuse with Extracellular Vesicles in Virus Neutralization Tests
- PMID: 38001893
- PMCID: PMC10669694
- DOI: 10.3390/biomedicines11112892
SARS-CoV-2 and Epstein-Barr Virus-like Particles Associate and Fuse with Extracellular Vesicles in Virus Neutralization Tests
Abstract
The successful development of effective viral vaccines depends on well-known correlates of protection, high immunogenicity, acceptable safety criteria, low reactogenicity, and well-designed immune monitoring and serology. Virus-neutralizing antibodies are often a good correlate of protective immunity, and their serum concentration is a key parameter during the pre-clinical and clinical testing of vaccine candidates. Viruses are inherently infectious and potentially harmful, but we and others developed replication-defective SARS-CoV-2 virus-like-particles (VLPs) as surrogates for infection to quantitate neutralizing antibodies with appropriate target cells using a split enzyme-based approach. Here, we show that SARS-CoV-2 and Epstein-Barr virus (EBV)-derived VLPs associate and fuse with extracellular vesicles in a highly specific manner, mediated by the respective viral fusion proteins and their corresponding host receptors. We highlight the capacity of virus-neutralizing antibodies to interfere with this interaction and demonstrate a potent application using this technology. To overcome the common limitations of most virus neutralization tests, we developed a quick in vitro diagnostic assay based on the fusion of SARS-CoV-2 VLPs with susceptible vesicles to quantitate neutralizing antibodies without the need for infectious viruses or living cells. We validated this method by testing a set of COVID-19 patient serum samples, correlated the results with those of a conventional test, and found good sensitivity and specificity. Furthermore, we demonstrate that this serological assay can be adapted to a human herpesvirus, EBV, and possibly other enveloped viruses.
Keywords: EBV; Epstein–Barr virus; SARS-CoV-2; antibody; diagnostic test; extracellular vesicle; fusion; virus neutralization test; virus-like particle.
Conflict of interest statement
Authors D.P., R.Z. and W.H. are listed as inventors on a patent application relating to virus neutralization assays jointly filed by Helmholtz Munich and Eximmium Biotechlogies (Munich, Germany). All other authors declare that they have no competing interest.
Figures
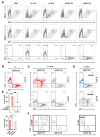
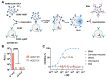
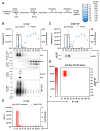
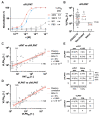
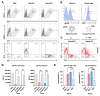
Similar articles
-
Quantitation of SARS-CoV-2 neutralizing antibodies with a virus-free, authentic test.PNAS Nexus. 2022 May;1(2):pgac045. doi: 10.1093/pnasnexus/pgac045. Epub 2022 Apr 14. PNAS Nexus. 2022. PMID: 36382127 Free PMC article.
-
A novel vaccine candidate based on chimeric virus-like particle displaying multiple conserved epitope peptides induced neutralizing antibodies against EBV infection.Theranostics. 2020 Apr 27;10(13):5704-5718. doi: 10.7150/thno.42494. eCollection 2020. Theranostics. 2020. PMID: 32483413 Free PMC article.
-
SARS-CoV-2 Neutralization in Convalescent Plasma and Commercial Lots of Plasma-Derived Immunoglobulin.BioDrugs. 2022 Jan;36(1):41-53. doi: 10.1007/s40259-021-00511-9. Epub 2021 Nov 29. BioDrugs. 2022. PMID: 34843105 Free PMC article.
-
An Assessment of Serological Assays for SARS-CoV-2 as Surrogates for Authentic Virus Neutralization.Microbiol Spectr. 2021 Oct 31;9(2):e0105921. doi: 10.1128/Spectrum.01059-21. Epub 2021 Oct 27. Microbiol Spectr. 2021. PMID: 34704832 Free PMC article.
-
Nanoparticle and virus-like particle vaccine approaches against SARS-CoV-2.J Microbiol. 2022 Mar;60(3):335-346. doi: 10.1007/s12275-022-1608-z. Epub 2022 Jan 28. J Microbiol. 2022. PMID: 35089583 Free PMC article. Review.
Cited by
-
Light-Induced Transformation of Virus-Like Particles on TiO2.ACS Appl Mater Interfaces. 2024 Jul 17;16(28):37275-37287. doi: 10.1021/acsami.4c07151. Epub 2024 Jul 3. ACS Appl Mater Interfaces. 2024. PMID: 38959130 Free PMC article.
References
-
- Khoury D.S., Cromer D., Reynaldi A., Schlub T.E., Wheatley A.K., Juno J.A., Subbarao K., Kent S.J., Triccas J.A., Davenport M.P. Neutralizing antibody levels are highly predictive of immune protection from symptomatic SARS-CoV-2 infection. Nat. Med. 2021;27:1205–1211. doi: 10.1038/s41591-021-01377-8. - DOI - PubMed
Grants and funding
LinkOut - more resources
Full Text Sources
Miscellaneous