This is a preprint.
CRISPR screens and lectin microarrays identify novel high mannose N-glycan regulators
- PMID: 37961200
- PMCID: PMC10634773
- DOI: 10.1101/2023.10.23.563662
CRISPR screens and lectin microarrays identify novel high mannose N-glycan regulators
Update in
-
CRISPR screens and lectin microarrays identify high mannose N-glycan regulators.Nat Commun. 2024 Nov 18;15(1):9970. doi: 10.1038/s41467-024-53225-1. Nat Commun. 2024. PMID: 39557836 Free PMC article.
Abstract
Glycans play critical roles in cellular signaling and function. Unlike proteins, glycan structures are not templated from genes but the concerted activity of many genes, making them historically challenging to study. Here, we present a strategy that utilizes pooled CRISPR screens and lectin microarrays to uncover and characterize regulators of cell surface glycosylation. We applied this approach to study the regulation of high mannose glycans - the starting structure of all asparagine(N)-linked-glycans. We used CRISPR screens to uncover the expanded network of genes controlling high mannose surface levels, followed by lectin microarrays to fully measure the complex effect of select regulators on glycosylation globally. Through this, we elucidated how two novel high mannose regulators - TM9SF3 and the CCC complex - control complex N-glycosylation via regulating Golgi morphology and function. Notably, this method allowed us to interrogate Golgi function in-depth and reveal that similar disruption to Golgi morphology can lead to drastically different glycosylation outcomes. Collectively, this work demonstrates a generalizable approach for systematically dissecting the regulatory network underlying glycosylation.
Figures
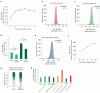
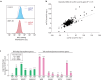
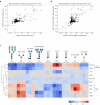
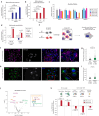
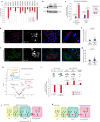
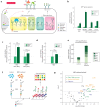
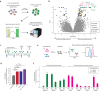
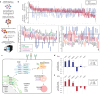
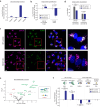
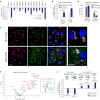
Similar articles
-
CRISPR screens and lectin microarrays identify high mannose N-glycan regulators.Nat Commun. 2024 Nov 18;15(1):9970. doi: 10.1038/s41467-024-53225-1. Nat Commun. 2024. PMID: 39557836 Free PMC article.
-
Depressing time: Waiting, melancholia, and the psychoanalytic practice of care.In: Kirtsoglou E, Simpson B, editors. The Time of Anthropology: Studies of Contemporary Chronopolitics. Abingdon: Routledge; 2020. Chapter 5. In: Kirtsoglou E, Simpson B, editors. The Time of Anthropology: Studies of Contemporary Chronopolitics. Abingdon: Routledge; 2020. Chapter 5. PMID: 36137063 Free Books & Documents. Review.
-
Using Experience Sampling Methodology to Capture Disclosure Opportunities for Autistic Adults.Autism Adulthood. 2023 Dec 1;5(4):389-400. doi: 10.1089/aut.2022.0090. Epub 2023 Dec 12. Autism Adulthood. 2023. PMID: 38116059 Free PMC article.
-
Unlocking data: Decision-maker perspectives on cross-sectoral data sharing and linkage as part of a whole-systems approach to public health policy and practice.Public Health Res (Southampt). 2024 Nov 20:1-30. doi: 10.3310/KYTW2173. Online ahead of print. Public Health Res (Southampt). 2024. PMID: 39582242
-
Timed intercourse for couples trying to conceive.Cochrane Database Syst Rev. 2023 Sep 15;9(9):CD011345. doi: 10.1002/14651858.CD011345.pub3. Cochrane Database Syst Rev. 2023. PMID: 37709293 Free PMC article. Review.
References
-
- Ohtsubo K. & Marth J. D. Glycosylation in cellular mechanisms of health and disease. Cell 126, 855–867 (2006). - PubMed
-
- Pinho S. S. & Reis C. A. Glycosylation in cancer: mechanisms and clinical implications. Nat. Rev. Cancer 15, 540–555 (2015). - PubMed
-
- Qin R. & Mahal L. K. The host glycomic response to pathogens. Curr. Opin. Struct. Biol. 68, 149–156 (2021). - PubMed
Publication types
Grants and funding
LinkOut - more resources
Full Text Sources
Research Materials