The Application of Graphene Field-Effect Transistor Biosensors in COVID-19 Detection Technology: A Review
- PMID: 37960464
- PMCID: PMC10650741
- DOI: 10.3390/s23218764
The Application of Graphene Field-Effect Transistor Biosensors in COVID-19 Detection Technology: A Review
Abstract
Coronavirus disease 2019 (COVID-19) is a disease caused by the infectious agent of severe acute respiratory syndrome coronavirus type 2 (SARS-CoV-2). The primary method of diagnosing SARS-CoV-2 is nucleic acid detection, but this method requires specialized equipment and is time consuming. Therefore, a sensitive, simple, rapid, and low-cost diagnostic test is needed. Graphene field-effect transistor (GFET) biosensors have become the most promising diagnostic technology for detecting SARS-CoV-2 due to their advantages of high sensitivity, fast-detection speed, label-free operation, and low detection limit. This review mainly focus on three types of GFET biosensors to detect SARS-CoV-2. GFET biosensors can quickly identify SARS-CoV-2 within ultra-low detection limits. Finally, we will outline the pros and cons of the diagnostic approaches as well as future directions.
Keywords: COVID-19; GFET biosensor; biological detection; biological sensors.
Conflict of interest statement
The authors declare no conflict of interest.
Figures
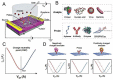
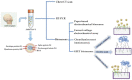
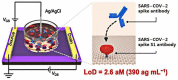
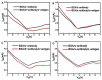
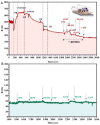
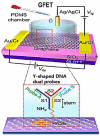
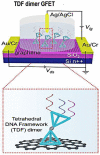
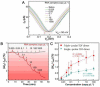
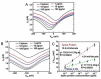
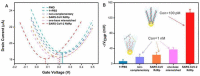
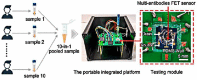
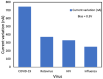
Similar articles
-
Graphene transistor-based biosensors for rapid detection of SARS-CoV-2.Bioelectrochemistry. 2024 Apr;156:108623. doi: 10.1016/j.bioelechem.2023.108623. Epub 2023 Dec 2. Bioelectrochemistry. 2024. PMID: 38070365
-
Rapid self-test of unprocessed viruses of SARS-CoV-2 and its variants in saliva by portable wireless graphene biosensor.Proc Natl Acad Sci U S A. 2022 Jul 12;119(28):e2206521119. doi: 10.1073/pnas.2206521119. Epub 2022 Jun 28. Proc Natl Acad Sci U S A. 2022. PMID: 35763566 Free PMC article.
-
Poly-l-Lysine-Modified Graphene Field-Effect Transistor Biosensors for Ultrasensitive Breast Cancer miRNAs and SARS-CoV-2 RNA Detection.Anal Chem. 2022 Jan 25;94(3):1626-1636. doi: 10.1021/acs.analchem.1c03786. Epub 2022 Jan 13. Anal Chem. 2022. PMID: 35025203
-
Graphene-based biosensors for detecting coronavirus: a brief review.Nanoscale. 2023 Nov 23;15(45):18184-18197. doi: 10.1039/d3nr04583h. Nanoscale. 2023. PMID: 37927083 Review.
-
Sensitivity-Enhancing Strategies of Graphene Field-Effect Transistor Biosensors for Biomarker Detection.ACS Sens. 2024 Jun 28;9(6):2705-2727. doi: 10.1021/acssensors.4c00322. Epub 2024 Jun 6. ACS Sens. 2024. PMID: 38843307 Review.
References
-
- Van Doremalen N., Bushmaker T., Morris D.H., Holbrook M.G., Gamble A., Williamson B.N., Tamin A., Harcourt J.L., Thornburg N.J., Gerber S.I., et al. Aerosol and Surface Stability of SARS-CoV-2 as Compared with SARS-CoV-1. N. Engl. J. Med. 2020;382:1564–1567. doi: 10.1056/NEJMc2004973. - DOI - PMC - PubMed
Publication types
MeSH terms
Substances
Grants and funding
LinkOut - more resources
Full Text Sources
Medical
Miscellaneous