Multi-Scale Imaging of the Dynamic Organization of Chromatin
- PMID: 37958958
- PMCID: PMC10649806
- DOI: 10.3390/ijms242115975
Multi-Scale Imaging of the Dynamic Organization of Chromatin
Abstract
Chromatin is now regarded as a heterogeneous and dynamic structure occupying a non-random position within the cell nucleus, where it plays a key role in regulating various functions of the genome. This current view of chromatin has emerged thanks to high spatiotemporal resolution imaging, among other new technologies developed in the last decade. In addition to challenging early assumptions of chromatin being regular and static, high spatiotemporal resolution imaging made it possible to visualize and characterize different chromatin structures such as clutches, domains and compartments. More specifically, super-resolution microscopy facilitates the study of different cellular processes at a nucleosome scale, providing a multi-scale view of chromatin behavior within the nucleus in different environments. In this review, we describe recent imaging techniques to study the dynamic organization of chromatin at high spatiotemporal resolution. We also discuss recent findings, elucidated by these techniques, on the chromatin landscape during different cellular processes, with an emphasis on the DNA damage response.
Keywords: DNA repair; chromatin organization and dynamics; high resolution imaging.
Conflict of interest statement
The authors declare no conflict of interest.
Figures
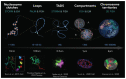
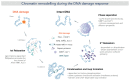
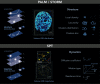
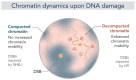
Similar articles
-
Chromatin behavior in living cells: Lessons from single-nucleosome imaging and tracking.Bioessays. 2022 Jul;44(7):e2200043. doi: 10.1002/bies.202200043. Epub 2022 Jun 3. Bioessays. 2022. PMID: 35661389 Review.
-
Advanced microscopy methods for visualizing chromatin structure.FEBS Lett. 2015 Oct 7;589(20 Pt A):3023-30. doi: 10.1016/j.febslet.2015.04.012. Epub 2015 Apr 17. FEBS Lett. 2015. PMID: 25896023 Review.
-
Dynamic Organization of Chromatin Domains Revealed by Super-Resolution Live-Cell Imaging.Mol Cell. 2017 Jul 20;67(2):282-293.e7. doi: 10.1016/j.molcel.2017.06.018. Epub 2017 Jul 14. Mol Cell. 2017. PMID: 28712725
-
Dynamic chromatin organization in the cell.Essays Biochem. 2019 Apr 23;63(1):133-145. doi: 10.1042/EBC20180054. Print 2019 Apr 23. Essays Biochem. 2019. PMID: 30967477 Review.
-
Condensed but liquid-like domain organization of active chromatin regions in living human cells.Sci Adv. 2023 Apr 5;9(14):eadf1488. doi: 10.1126/sciadv.adf1488. Epub 2023 Apr 5. Sci Adv. 2023. PMID: 37018405 Free PMC article.
References
-
- Chi K.R. Super-Resolution Microscopy: Breaking the Limits. Nat. Methods. 2009;6:15–18. doi: 10.1038/nmeth.f.234. - DOI
Publication types
MeSH terms
Substances
Grants and funding
LinkOut - more resources
Full Text Sources