In situ quantification of osmotic pressure within living embryonic tissues
- PMID: 37919265
- PMCID: PMC10622550
- DOI: 10.1038/s41467-023-42024-9
In situ quantification of osmotic pressure within living embryonic tissues
Abstract
Mechanics is known to play a fundamental role in many cellular and developmental processes. Beyond active forces and material properties, osmotic pressure is believed to control essential cell and tissue characteristics. However, it remains very challenging to perform in situ and in vivo measurements of osmotic pressure. Here we introduce double emulsion droplet sensors that enable local measurements of osmotic pressure intra- and extra-cellularly within 3D multicellular systems, including living tissues. After generating and calibrating the sensors, we measure the osmotic pressure in blastomeres of early zebrafish embryos as well as in the interstitial fluid between the cells of the blastula by monitoring the size of droplets previously inserted in the embryo. Our results show a balance between intracellular and interstitial osmotic pressures, with values of approximately 0.7 MPa, but a large pressure imbalance between the inside and outside of the embryo. The ability to measure osmotic pressure in 3D multicellular systems, including developing embryos and organoids, will help improve our understanding of its role in fundamental biological processes.
© 2023. The Author(s).
Conflict of interest statement
A.V. and O.C. declare the following competing interests: Provisional patent, application number 63/383,647, Systems and Methods for Measuring Osmotic Pressure, Antoine Vian and Otger Campas, 2022. There are no other competing interests for any of the authors.
Figures
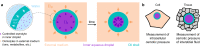
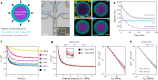
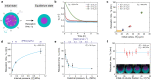
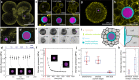
Similar articles
-
Design of double emulsions by osmotic pressure tailoring.Langmuir. 2004 Apr 27;20(9):3574-82. doi: 10.1021/la036396k. Langmuir. 2004. PMID: 15875386
-
Evolution of osmotic pressure in solid tumors.J Biomech. 2014 Nov 7;47(14):3441-7. doi: 10.1016/j.jbiomech.2014.09.019. Epub 2014 Sep 28. J Biomech. 2014. PMID: 25287111 Free PMC article.
-
The jamming elasticity of emulsions stabilized by ionic surfactants.Soft Matter. 2014 Jul 28;10(28):5040-4. doi: 10.1039/c4sm00389f. Soft Matter. 2014. PMID: 24913542
-
A toolbox to explore the mechanics of living embryonic tissues.Semin Cell Dev Biol. 2016 Jul;55:119-30. doi: 10.1016/j.semcdb.2016.03.011. Epub 2016 Apr 6. Semin Cell Dev Biol. 2016. PMID: 27061360 Free PMC article. Review.
-
Interstitial-lymphatic mechanisms in the control of extracellular fluid volume.Physiol Rev. 1993 Jan;73(1):1-78. doi: 10.1152/physrev.1993.73.1.1. Physiol Rev. 1993. PMID: 8419962 Review.
Cited by
-
Quantifying turgor pressure in budding and fission yeasts based upon osmotic properties.bioRxiv [Preprint]. 2023 Oct 2:2023.06.07.544129. doi: 10.1101/2023.06.07.544129. bioRxiv. 2023. Update in: Mol Biol Cell. 2023 Dec 1;34(13):ar133. doi: 10.1091/mbc.E23-06-0215 PMID: 37333400 Free PMC article. Updated. Preprint.
-
Push and pull: how to measure the forces that sculpt embryos.Nature. 2024 Jun;630(8017):780-782. doi: 10.1038/d41586-024-02029-w. Nature. 2024. PMID: 38886553 No abstract available.
-
Osmotic Pressure and Its Biological Implications.Int J Mol Sci. 2024 Mar 14;25(6):3310. doi: 10.3390/ijms25063310. Int J Mol Sci. 2024. PMID: 38542282 Free PMC article. Review.
-
Small hand-designed convolutional neural networks outperform transfer learning in automated cell shape detection in confluent tissues.PLoS One. 2023 Feb 16;18(2):e0281931. doi: 10.1371/journal.pone.0281931. eCollection 2023. PLoS One. 2023. PMID: 36795738 Free PMC article.
-
Quantifying turgor pressure in budding and fission yeasts based upon osmotic properties.Mol Biol Cell. 2023 Dec 1;34(13):ar133. doi: 10.1091/mbc.E23-06-0215. Epub 2023 Oct 30. Mol Biol Cell. 2023. PMID: 37903220 Free PMC article.
References
Publication types
MeSH terms
Substances
Grants and funding
LinkOut - more resources
Full Text Sources