Carbon Nanomaterial-Based Hydrogels as Scaffolds in Tissue Engineering: A Comprehensive Review
- PMID: 37915750
- PMCID: PMC10616695
- DOI: 10.2147/IJN.S436867
Carbon Nanomaterial-Based Hydrogels as Scaffolds in Tissue Engineering: A Comprehensive Review
Abstract
Carbon-based nanomaterials (CBNs) are a category of nanomaterials with various systems based on combinations of sp2 and sp3 hybridized carbon bonds, morphologies, and functional groups. CBNs can exhibit distinguished properties such as high mechanical strength, chemical stability, high electrical conductivity, and biocompatibility. These desirable physicochemical properties have triggered their uses in many fields, including biomedical applications. In this review, we specifically focus on applying CBNs as scaffolds in tissue engineering, a therapeutic approach whereby CBNs can act for the regeneration or replacement of damaged tissue. Here, an overview of the structures and properties of different CBNs will first be provided. We will then discuss state-of-the-art advancements of CBNs and hydrogels as scaffolds for regenerating various types of human tissues. Finally, a perspective of future potentials and challenges in this field will be presented. Since this is a very rapidly growing field, we expect that this review will promote interdisciplinary efforts in developing effective tissue regeneration scaffolds for clinical applications.
Keywords: biomaterial; carbon; nanotechnology; scaffold; tissue engineering.
© 2023 Stocco et al.
Conflict of interest statement
The authors report no conflicts of interest in this work.
Figures
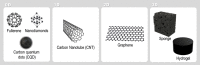
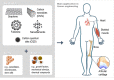
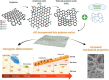
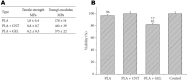
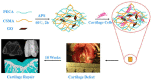
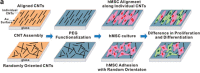
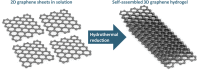
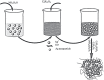
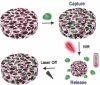
Similar articles
-
Nanocellulose-based hydrogels as versatile materials with interesting functional properties for tissue engineering applications.J Mater Chem B. 2024 Aug 14;12(32):7692-7759. doi: 10.1039/d4tb00397g. J Mater Chem B. 2024. PMID: 38805188 Review.
-
Nanomaterial-Based Electrically Conductive Hydrogels for Cardiac Tissue Repair.Int J Nanomedicine. 2022 Dec 9;17:6181-6200. doi: 10.2147/IJN.S386763. eCollection 2022. Int J Nanomedicine. 2022. PMID: 36531116 Free PMC article. Review.
-
Advances in Carbon Based Nanomaterials for Bio-Medical Applications.Curr Med Chem. 2019;26(38):6851-6877. doi: 10.2174/0929867326666181126113605. Curr Med Chem. 2019. PMID: 30474523 Review.
-
Biocompatibility of hydrogel-based scaffolds for tissue engineering applications.Biotechnol Adv. 2017 Sep;35(5):530-544. doi: 10.1016/j.biotechadv.2017.05.006. Epub 2017 May 27. Biotechnol Adv. 2017. PMID: 28558979 Review.
-
Synthetic peptide hydrogels as 3D scaffolds for tissue engineering.Adv Drug Deliv Rev. 2020;160:78-104. doi: 10.1016/j.addr.2020.10.005. Epub 2020 Oct 19. Adv Drug Deliv Rev. 2020. PMID: 33091503
Cited by
-
Evolution of Hybrid Hydrogels: Next-Generation Biomaterials for Drug Delivery and Tissue Engineering.Gels. 2024 Mar 22;10(4):216. doi: 10.3390/gels10040216. Gels. 2024. PMID: 38667635 Free PMC article. Review.
References
-
- Feng W, Zhu X, Li F. Recent advances in the optimization and functionalization of upconversion nanomaterials for in vivo bioapplications. NPG Asia Mater. 2013;5(12):e75–e75. doi:10.1038/am.2013.63 - DOI
Publication types
MeSH terms
Substances
LinkOut - more resources
Full Text Sources