Septins modulate the autophagy response after nutrient starvation
- PMID: 37910217
- PMCID: PMC10881159
- DOI: 10.1091/mbc.E22-11-0520
Septins modulate the autophagy response after nutrient starvation
Abstract
The pathways that induce macroautophagy (referred to as autophagy hereafter) in response to the stress of starvation are well conserved and essential under nutrient-limiting conditions. However, less is understood about the mechanisms that modulate the autophagy response. Here we present evidence that after induction of autophagy in budding yeast septin filaments rapidly assemble into discrete patches distributed along the cell cortex. These patches gradually mature over 12 h of nutrient deprivation to form extended structures around Atg9 membranes tethered at the cortical endoplasmic reticulum, a class of membranes that are limiting for autophagosome biogenesis. Loss of cortical septin structures alters the kinetics of autophagy activation and most dramatically extends the duration of the autophagy response. In wild-type cells, diffusion of Atg9 membranes at the cell cortex undergoes transient pauses that are dependent on septins, and septins at the bud neck block the diffusion of Atg9 membranes between mother and daughter cells. We conclude that septins reorganize at the cell cortex during autophagy to locally limit access of Atg9 membranes to autophagosome assembly sites, and thus modulate the autophagy response during nutrient deprivation.
Figures
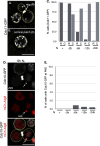
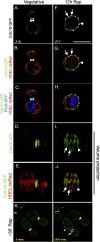
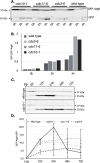
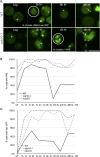
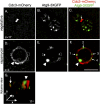
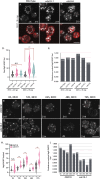
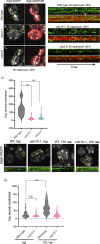
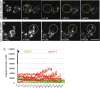
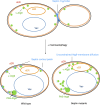
Similar articles
-
Septins are involved at the early stages of macroautophagy in S. cerevisiae.J Cell Sci. 2018 Feb 22;131(4):jcs209098. doi: 10.1242/jcs.209098. J Cell Sci. 2018. PMID: 29361537 Free PMC article.
-
Dynamics of septin ring and collar formation in Saccharomyces cerevisiae.Biol Chem. 2011 Aug;392(8-9):689-97. doi: 10.1515/BC.2011.075. Epub 2011 Jul 7. Biol Chem. 2011. PMID: 21736496 Free PMC article.
-
--Atg9 interactions via its transmembrane domains are required for phagophore expansion during autophagy.Autophagy. 2023 May;19(5):1459-1478. doi: 10.1080/15548627.2022.2136340. Epub 2022 Nov 10. Autophagy. 2023. PMID: 36354155 Free PMC article.
-
Septin localization and function during autophagy.Curr Genet. 2018 Oct;64(5):1037-1041. doi: 10.1007/s00294-018-0834-8. Epub 2018 Apr 12. Curr Genet. 2018. PMID: 29651536 Review.
-
Autophagosome biogenesis comes out of the black box.Nat Cell Biol. 2021 May;23(5):450-456. doi: 10.1038/s41556-021-00669-y. Epub 2021 Apr 26. Nat Cell Biol. 2021. PMID: 33903736 Free PMC article. Review.
References
-
- Barve G, Sanyal P, Manjithaya R (2018a). Septin localization and function during autophagy. Curr Genet 64, 1037–1041. - PubMed
MeSH terms
Substances
LinkOut - more resources
Full Text Sources
Molecular Biology Databases