Deep Learning-Predicted Dihydroartemisinin Rescues Osteoporosis by Maintaining Mesenchymal Stem Cell Stemness through Activating Histone 3 Lys 9 Acetylation
- PMID: 37901168
- PMCID: PMC10604014
- DOI: 10.1021/acscentsci.3c00794
Deep Learning-Predicted Dihydroartemisinin Rescues Osteoporosis by Maintaining Mesenchymal Stem Cell Stemness through Activating Histone 3 Lys 9 Acetylation
Abstract
Maintaining the stemness of bone marrow mesenchymal stem cells (BMMSCs) is crucial for bone homeostasis and regeneration. However, in vitro expansion and bone diseases impair BMMSC stemness, limiting its functionality in bone tissue engineering. Using a deep learning-based efficacy prediction system and bone tissue sequencing, we identify a natural small-molecule compound, dihydroartemisinin (DHA), that maintains BMMSC stemness and enhances bone regeneration. During long-term in vitro expansion, DHA preserves BMMSC stemness characteristics, including its self-renewal ability and unbiased differentiation. In an osteoporosis mouse model, oral administration of DHA restores the femur trabecular structure, bone density, and BMMSC stemness in situ. Mechanistically, DHA maintains BMMSC stemness by promoting histone 3 lysine 9 acetylation via GCN5 activation both in vivo and in vitro. Furthermore, the bone-targeted delivery of DHA by mesoporous silica nanoparticles improves its therapeutic efficacy in osteoporosis. Collectively, DHA could be a promising therapeutic agent for treating osteoporosis by maintaining BMMSC stemness.
© 2023 The Authors. Published by American Chemical Society.
Conflict of interest statement
The authors declare no competing financial interest.
Figures
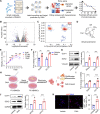
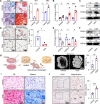
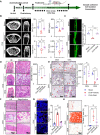
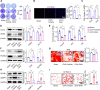
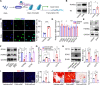
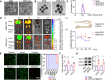
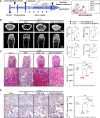
Similar articles
-
Melatonin Treatment Improves Mesenchymal Stem Cells Therapy by Preserving Stemness during Long-term In Vitro Expansion.Theranostics. 2016 Aug 8;6(11):1899-917. doi: 10.7150/thno.15412. eCollection 2016. Theranostics. 2016. PMID: 27570559 Free PMC article.
-
Transplantation of mesenchymal stem cells ameliorates secondary osteoporosis through interleukin-17-impaired functions of recipient bone marrow mesenchymal stem cells in MRL/lpr mice.Stem Cell Res Ther. 2015 May 27;6(1):104. doi: 10.1186/s13287-015-0091-4. Stem Cell Res Ther. 2015. PMID: 26012584 Free PMC article.
-
Improvement in viability and mineralization of osteoporotic bone marrow mesenchymal stem cell through combined application of photobiomodulation therapy and oxytocin.Lasers Med Sci. 2020 Apr;35(3):557-566. doi: 10.1007/s10103-019-02848-8. Epub 2019 Aug 9. Lasers Med Sci. 2020. PMID: 31399862
-
Differentiation of Bone Marrow Mesenchymal Stem Cells in Osteoblasts and Adipocytes and its Role in Treatment of Osteoporosis.Med Sci Monit. 2016 Jan 21;22:226-33. doi: 10.12659/msm.897044. Med Sci Monit. 2016. PMID: 26795027 Free PMC article. Review.
-
Bone marrow mesenchymal stem cells: fat on and blast off by FGF21.Int J Biochem Cell Biol. 2013 Mar;45(3):546-9. doi: 10.1016/j.biocel.2012.12.014. Epub 2012 Dec 25. Int J Biochem Cell Biol. 2013. PMID: 23270727 Free PMC article. Review.
Cited by
-
From Genomics to Metabolomics: Molecular Insights into Osteoporosis for Enhanced Diagnostic and Therapeutic Approaches.Biomedicines. 2024 Oct 18;12(10):2389. doi: 10.3390/biomedicines12102389. Biomedicines. 2024. PMID: 39457701 Free PMC article. Review.
-
Artificial Intelligence (AI): A Potential Game Changer in Regenerative Orthopedics-A Scoping Review.Indian J Orthop. 2024 Jun 2;58(10):1362-1374. doi: 10.1007/s43465-024-01189-1. eCollection 2024 Oct. Indian J Orthop. 2024. PMID: 39324081 Review.
-
Deep Learning and Drug Discovery for Healthy Aging.ACS Cent Sci. 2023 Oct 17;9(10):1860-1863. doi: 10.1021/acscentsci.3c01212. eCollection 2023 Oct 25. ACS Cent Sci. 2023. PMID: 37901176 Free PMC article. No abstract available.
References
LinkOut - more resources
Full Text Sources