A Bidens pilosa L. Non-Polar Extract Modulates the Polarization of Human Macrophages and Dendritic Cells into an Anti-Inflammatory Phenotype
- PMID: 37894572
- PMCID: PMC10608814
- DOI: 10.3390/molecules28207094
A Bidens pilosa L. Non-Polar Extract Modulates the Polarization of Human Macrophages and Dendritic Cells into an Anti-Inflammatory Phenotype
Abstract
Different communities around the world traditionally use Bidens pilosa L. for medicinal purposes, mainly for its anti-inflammatory, antinociceptive, and antioxidant properties; it is used as an ingredient in teas or herbal medicines for the treatment of pain, inflammation, and immunological disorders. Several studies have been conducted that prove the immunomodulatory properties of this plant; however, it is not known whether the immunomodulatory properties of B. pilosa are mediated by its ability to modulate antigen-presenting cells (APCs) such as macrophages (MØs) and dendritic cells (DCs) (through polarization or the maturation state, respectively). Different polar and non-polar extracts and fractions were prepared from the aerial part of B. pilosa. Their cytotoxic and immunomodulatory effects were first tested on human peripheral blood mononuclear cells (PBMCs) and phytohemagglutinin (PHA)-stimulated PBMCs, respectively, via an MTT assay. Then, the non-cytotoxic plant extracts and fractions that showed the highest immunomodulatory activity were selected to evaluate their effects on human MØ polarization and DC maturation (cell surface phenotype and cytokine secretion) through multiparametric flow cytometry. Finally, the chemical compounds of the B. pilosa extract that showed the most significant immunomodulatory effects on human APCs were identified using gas chromatography coupled with mass spectrometry. The petroleum ether extract and the ethyl acetate and hydroalcoholic fractions obtained from B. pilosa showed low cytotoxicity and modulated the PHA-stimulated proliferation of PBMCs. Furthermore, the B. pilosa petroleum ether extract induced M2 polarization or a hybrid M1/M2 phenotype in MØs and a semi-mature status in DCs, regardless of exposure to a maturation stimulus. The immunomodulatory activity of the non-polar (petroleum ether) extract of B. pilosa on human PBMC proliferation, M2 polarization of MØs, and semi-mature status in DCs might be attributed to the low-medium polarity components in the extract, such as phytosterol terpenes and fatty acid esters.
Keywords: Bidens pilosa L.; dendritic cells; immunomodulation; macrophages; phytochemistry; phytotherapy; traditional medicine.
Conflict of interest statement
The authors declare no conflict of interest.
Figures
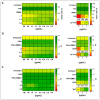
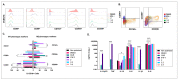

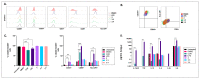
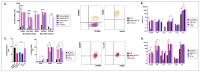
Similar articles
-
Anticancer effect of petroleum ether extract from Bidens pilosa L and its constituent's analysis by GC-MS.J Ethnopharmacol. 2018 May 10;217:126-133. doi: 10.1016/j.jep.2018.02.019. Epub 2018 Feb 15. J Ethnopharmacol. 2018. PMID: 29454912
-
Pharmacological potential of Bidens pilosa L. and determination of bioactive compounds using UHPLC-QqQLIT-MS/MS and GC/MS.BMC Complement Altern Med. 2017 Nov 16;17(1):492. doi: 10.1186/s12906-017-2000-0. BMC Complement Altern Med. 2017. PMID: 29145848 Free PMC article.
-
Immunomodulatory Properties of Natural Extracts and Compounds Derived from Bidens pilosa L.: Literature Review.Pharmaceutics. 2023 May 13;15(5):1491. doi: 10.3390/pharmaceutics15051491. Pharmaceutics. 2023. PMID: 37242733 Free PMC article. Review.
-
Analgesic and antiinflammatory activities of the ethyl acetate fraction of Bidens pilosa (Asteraceae).Inflammopharmacology. 2014 Apr;22(2):105-14. doi: 10.1007/s10787-013-0196-2. Epub 2013 Nov 16. Inflammopharmacology. 2014. PMID: 24242914
-
Compilation of secondary metabolites from Bidens pilosa L.Molecules. 2011 Jan 26;16(2):1070-102. doi: 10.3390/molecules16021070. Molecules. 2011. PMID: 21270729 Free PMC article. Review.
References
-
- Gea-Banacloche J.C. Immunomodulation. In: Runge M.S., Patterson C., editors. Principles of Molecular Medicine. Humana Press; Totowa, NJ, USA: 2006. pp. 893–904. - DOI
MeSH terms
Substances
Grants and funding
LinkOut - more resources
Full Text Sources
Miscellaneous