ATG9 resides on a unique population of small vesicles in presynaptic nerve terminals
- PMID: 37881948
- PMCID: PMC11062364
- DOI: 10.1080/15548627.2023.2274204
ATG9 resides on a unique population of small vesicles in presynaptic nerve terminals
Abstract
In neurons, autophagosome biogenesis occurs mainly in distal axons, followed by maturation during retrograde transport. Autophagosomal growth depends on the supply of membrane lipids which requires small vesicles containing ATG9, a lipid scramblase essential for macroautophagy/autophagy. Here, we show that ATG9-containing vesicles are enriched in synapses and resemble synaptic vesicles in size and density. The proteome of ATG9-containing vesicles immuno-isolated from nerve terminals showed conspicuously low levels of trafficking proteins except of the AP2-complex and some enzymes involved in endosomal phosphatidylinositol metabolism. Super resolution microscopy of nerve terminals and isolated vesicles revealed that ATG9-containing vesicles represent a distinct vesicle population with limited overlap not only with synaptic vesicles but also other membranes of the secretory pathway, uncovering a surprising heterogeneity in their membrane composition. Our results are compatible with the view that ATG9-containing vesicles function as lipid shuttles that scavenge membrane lipids from various intracellular membranes to support autophagosome biogenesis.Abbreviations: AP: adaptor related protein complex: ATG2: autophagy related 2; ATG9: autophagy related 9; DNA PAINT: DNA-based point accumulation for imaging in nanoscale topography; DyMIN STED: dynamic minimum stimulated emission depletion; EL: endosome and lysosome; ER: endoplasmic reticulum; GA: Golgi apparatus; iBAQ: intensity based absolute quantification; LAMP: lysosomal-associated membrane protein; M6PR: mannose-6-phosphate receptor, cation dependent; Minflux: minimal photon fluxes; Mito: mitochondria; MS: mass spectrometry; PAS: phagophore assembly site; PM: plasma membrane; Px: peroxisome; RAB26: RAB26, member RAS oncogene family; RAB3A: RAB3A, member RAS oncogene family; RAB5A: RAB5A, member RAS oncogene family; SNARE: soluble N-ethylmaleimide-sensitive-factor attachment receptor; SVs: synaptic vesicles; SYP: synaptophysin; TGN: trans-Golgi network; TRAPP: transport protein particle; VTI1: vesicle transport through interaction with t-SNAREs.
Keywords: ATG9; RAB26; autophagy; synapse; synaptic vesicles; vesicle proteome.
Conflict of interest statement
No potential conflict of interest was reported by the author(s).
Figures
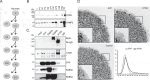
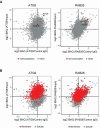
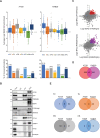
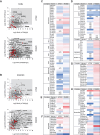
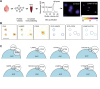
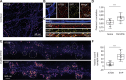
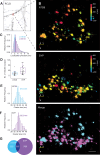
Similar articles
-
--Atg9 interactions via its transmembrane domains are required for phagophore expansion during autophagy.Autophagy. 2023 May;19(5):1459-1478. doi: 10.1080/15548627.2022.2136340. Epub 2022 Nov 10. Autophagy. 2023. PMID: 36354155 Free PMC article.
-
VAMP724 and VAMP726 are involved in autophagosome formation in Arabidopsis thaliana.Autophagy. 2023 May;19(5):1406-1423. doi: 10.1080/15548627.2022.2127240. Epub 2022 Oct 13. Autophagy. 2023. PMID: 36130166 Free PMC article.
-
The SNARE Vti1a-beta is localized to small synaptic vesicles and participates in a novel SNARE complex.J Neurosci. 2000 Aug 1;20(15):5724-32. doi: 10.1523/JNEUROSCI.20-15-05724.2000. J Neurosci. 2000. PMID: 10908612 Free PMC article.
-
TBC1D14 sets the TRAPP for ATG9.Autophagy. 2016 Jul 2;12(7):1212-3. doi: 10.1080/15548627.2016.1177696. Epub 2016 May 12. Autophagy. 2016. PMID: 27171758 Free PMC article. Review.
-
TRP Channel Trafficking.In: Liedtke WB, Heller S, editors. TRP Ion Channel Function in Sensory Transduction and Cellular Signaling Cascades. Boca Raton (FL): CRC Press/Taylor & Francis; 2007. Chapter 23. In: Liedtke WB, Heller S, editors. TRP Ion Channel Function in Sensory Transduction and Cellular Signaling Cascades. Boca Raton (FL): CRC Press/Taylor & Francis; 2007. Chapter 23. PMID: 21204515 Free Books & Documents. Review.
Cited by
-
Monitoring of activity-driven trafficking of endogenous synaptic proteins through proximity labeling.PLoS Biol. 2024 Oct 28;22(10):e3002860. doi: 10.1371/journal.pbio.3002860. eCollection 2024 Oct. PLoS Biol. 2024. PMID: 39466808 Free PMC article.
-
Plekhg5 controls the unconventional secretion of Sod1 by presynaptic secretory autophagy.Nat Commun. 2024 Oct 4;15(1):8622. doi: 10.1038/s41467-024-52875-5. Nat Commun. 2024. PMID: 39366938 Free PMC article.
-
Autophagy: Are Amino Acid Signals Dependent on the mTORC1 Pathway or Independent?Curr Issues Mol Biol. 2024 Aug 13;46(8):8780-8793. doi: 10.3390/cimb46080519. Curr Issues Mol Biol. 2024. PMID: 39194736 Free PMC article. Review.
-
Absence of ATG9A and synaptophysin demixing on Rab5 mutation-induced giant endosomes.Mol Brain. 2024 Sep 2;17(1):63. doi: 10.1186/s13041-024-01132-3. Mol Brain. 2024. PMID: 39223639 Free PMC article.
-
Emerging roles of ATG9/ATG9A in autophagy: implications for cell and neurobiology.Autophagy. 2024 Nov;20(11):2373-2387. doi: 10.1080/15548627.2024.2384349. Epub 2024 Aug 4. Autophagy. 2024. PMID: 39099167 Free PMC article. Review.
References
Publication types
MeSH terms
Substances
Grants and funding
LinkOut - more resources
Full Text Sources
Other Literature Sources
Research Materials
Miscellaneous